
Part:BBa_K525224
S-layer cspB from Corynebacterium halotolerans with TAT-sequence, PT7 and RBS
S-layers (crystalline bacterial surface layer) are crystal-like layers consisting of multiple protein monomers and can be found in various (archae-)bacteria. They constitute the outermost part of the cell wall. Especially their ability for self-assembly into distinct geometries is of scientific interest. At phase boundaries, in solutions and on a variety of surfaces they form different lattice structures. The geometry and arrangement is determined by the C-terminal self assembly-domain, which is specific for each S-layer protein. The most common lattice geometries are oblique, square and hexagonal. By modifying the characteristics of the S-layer through combination with functional groups and protein domains as well as their defined position and orientation to eachother (determined by the S-layer geometry) it is possible to realize various practical applications ([http://onlinelibrary.wiley.com/doi/10.1111/j.1574-6968.2006.00573.x/full Sleytr et al., 2007]).
Usage and Biology
S-layer proteins can be used as scaffold for nanobiotechnological applications and devices by e.g. fusing the S-layer's self-assembly domain to other functional protein domains. It is possible to coat surfaces and liposomes with S-layers. A big advantage of S-layers: after expressing in E. coli and purification, the nanobiotechnological system is cell-free. This enhances the biological security of a device.
Important parameters
Experiment | Characteristic | Result |
---|---|---|
Expression (E. coli) | Compatibility | E. coli KRX |
Induction of expression | L-rhamnose for induction of T7 polymerase | |
Specific growth rate (un-/induced) | 0.251 h-1 / 0.157 h-1 | |
Doubling time (un-/induced) | 2.76 h / 4.42 h | |
Characterization | ||
Number of amino acids | 459 | |
Molecular weight | 50.6 kDa | |
Theoretical pI | 4.25 | |
Localization | mainly cell membrane | |
partially culture supernatant | ||
partially cytoplasm | ||
not periplasm |
Sequence and Features
- 10INCOMPATIBLE WITH RFC[10]Illegal PstI site found at 440
- 12INCOMPATIBLE WITH RFC[12]Illegal PstI site found at 440
- 21INCOMPATIBLE WITH RFC[21]Illegal BglII site found at 1191
Illegal XhoI site found at 647 - 23INCOMPATIBLE WITH RFC[23]Illegal PstI site found at 440
- 25INCOMPATIBLE WITH RFC[25]Illegal PstI site found at 440
Illegal NgoMIV site found at 314
Illegal NgoMIV site found at 1403
Illegal AgeI site found at 89
Illegal AgeI site found at 305
Illegal AgeI site found at 546
Illegal AgeI site found at 593 - 1000INCOMPATIBLE WITH RFC[1000]Illegal BsaI site found at 995
Illegal BsaI.rc site found at 302
Illegal BsaI.rc site found at 680
Illegal BsaI.rc site found at 1082
Expression in E. coli
For characterization, the modified cspB gene was fused to a monomeric RFP (BBa_E1010) using Gibson assembly.
The CspB| mRFP fusion protein was overexpressed in E. coli KRX after induction of a T7 polymerase gene in the KRX's genome by supplementation of 0.1 % L-rhamnose using the autinduction protocol developed by Promega.
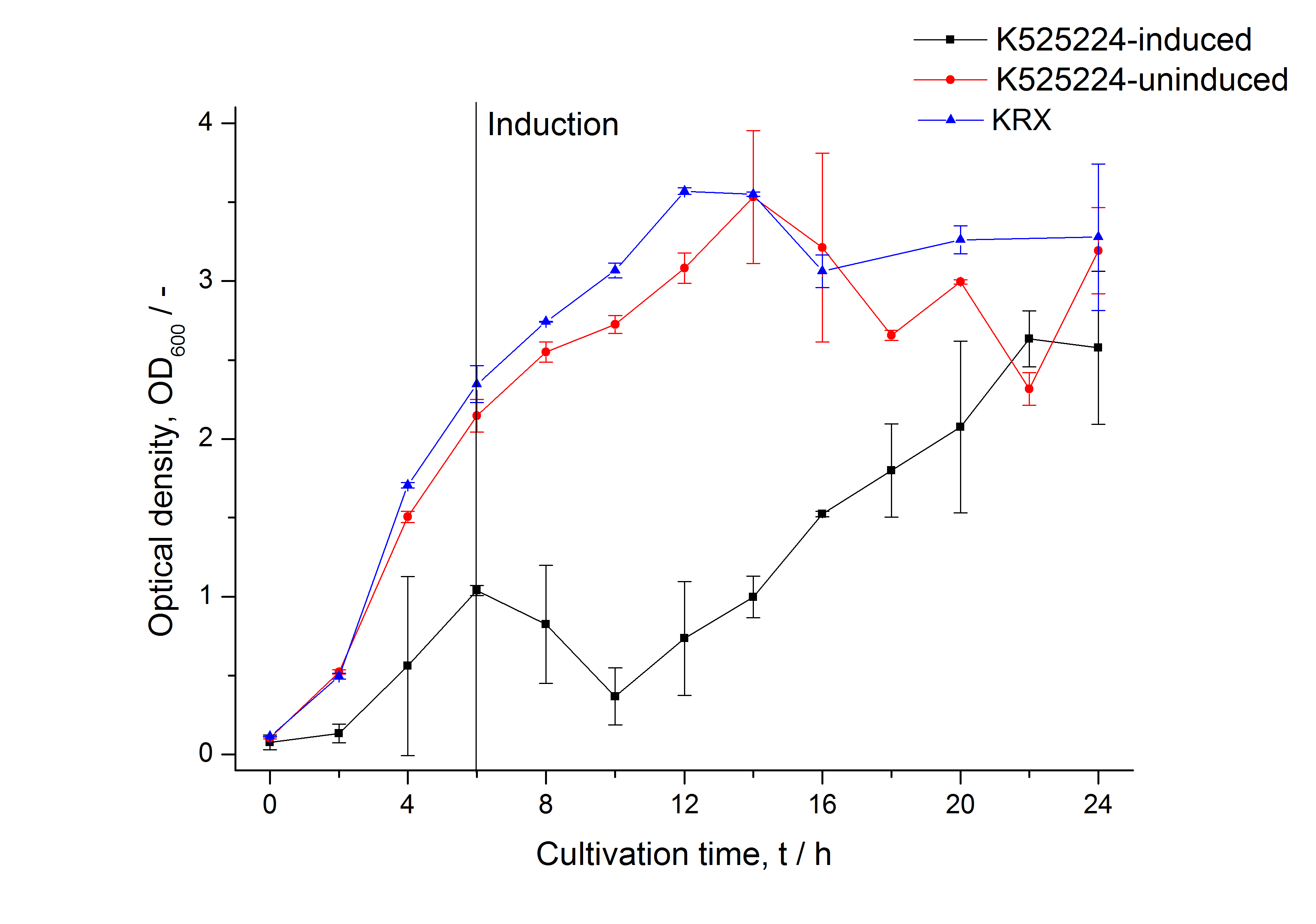
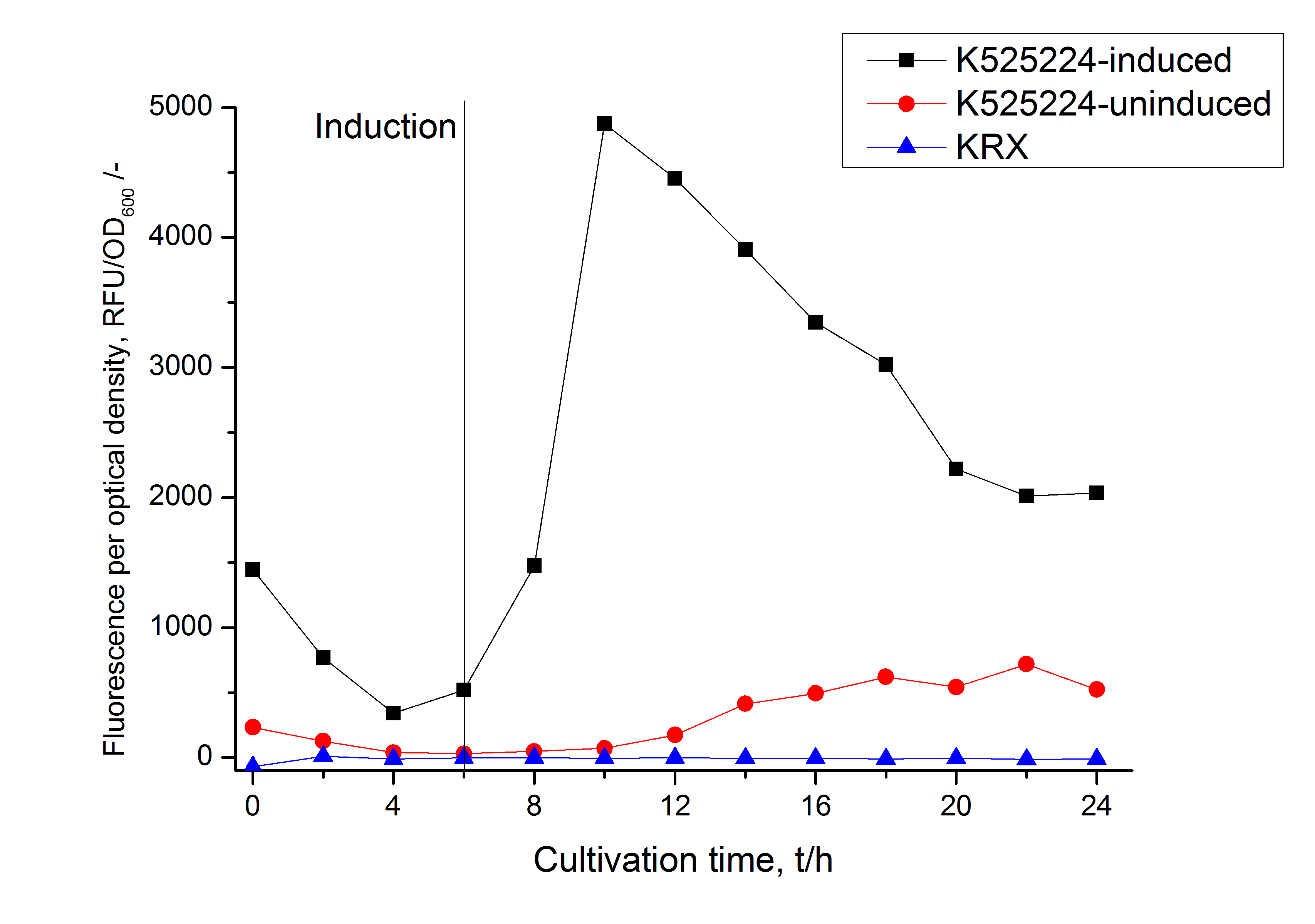
Identification and localisation
After a cultivation time of 18 h the mRFP|CspB the localization of the fusion protein in E. coli KRX was analyzed. Therefore a part of the produced biomass was mechanically disrupted and the resulting lysate was washed with ddH2O. Then the lysate was treated with ionic, nonionic and zwitterionic detergents to release the mRFP|CspB out of the membranes, if it integrates. From the other part of the cells the periplasm was detached by using an osmotic shock. The existance of fluorescene in the periplasm fraction, showed in fig. 3, indicates that C. halotolerans TAT-signal sequence is at least in part functional in E. coli KRX.
Specific for BBa_K525224 fused with mRFP is the proportional to the mRFP fusion proteins of K525222 and K525223 high fluorescence in the culture supernatant. This indicates that the fusion protein is secreted into the periplasm via the TAT-pathway and partly released into the culture medium. Because there is no known release pathway for S-layer proteins in E. coli the periplasm might burst in consequence of the overexpression.
The fluorescence in all cultivation fractions plus the fluorescence in the lysis und wash fraction shows that the fusion protein is water soluble and doesn't sediment during centrifugation.
The absence of fluorescence indicates that the expressed fusion protein doesn't form inclusion bodies during cultivation.
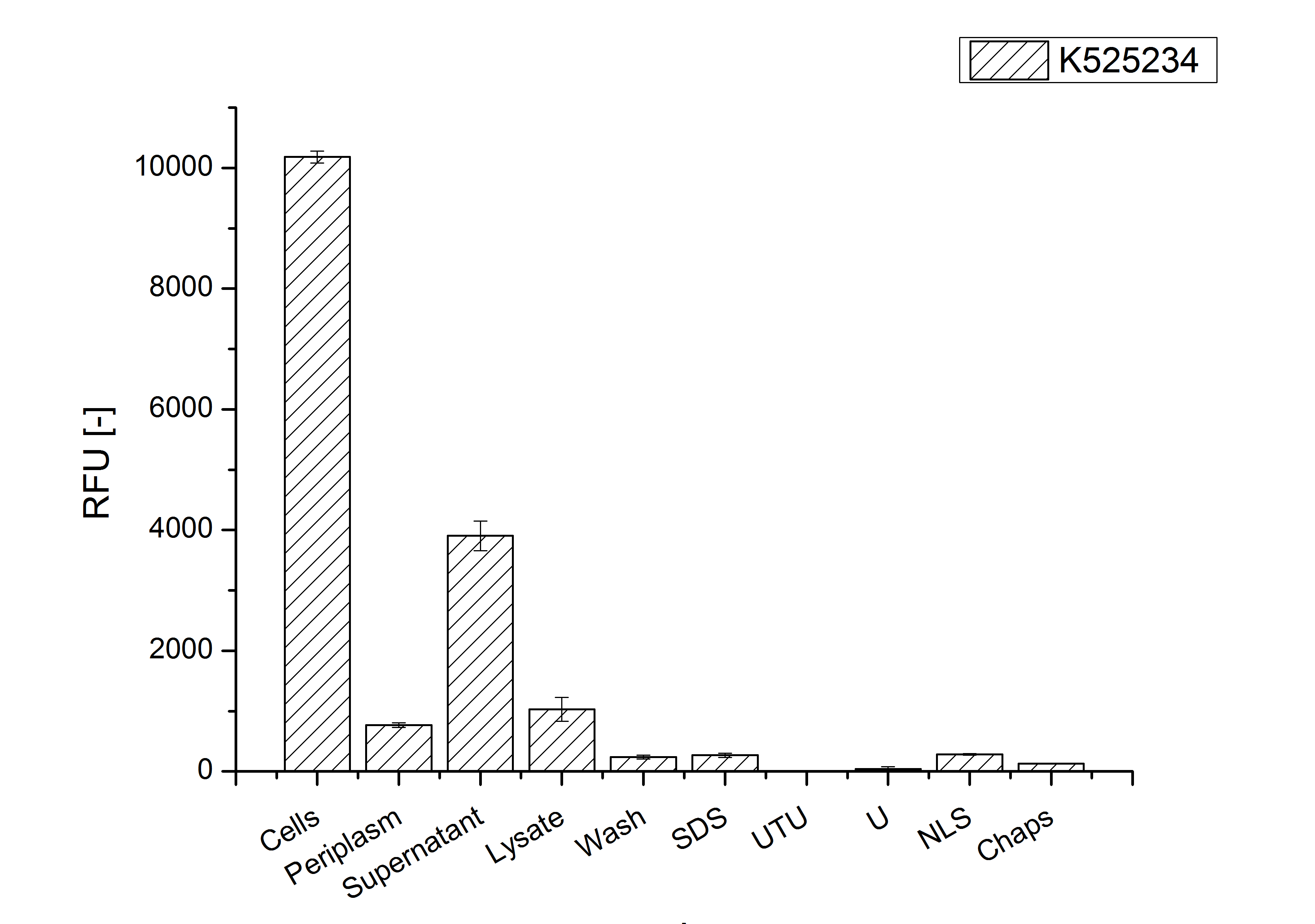
To obtain specific information about the location of the S-layer fusion protein, after comparison with same treated fraction of E. coli KRX all gel bands in a defined size area were cut out of the gel and analysed with MALDI-TOF. Results are shown in fig. 4.
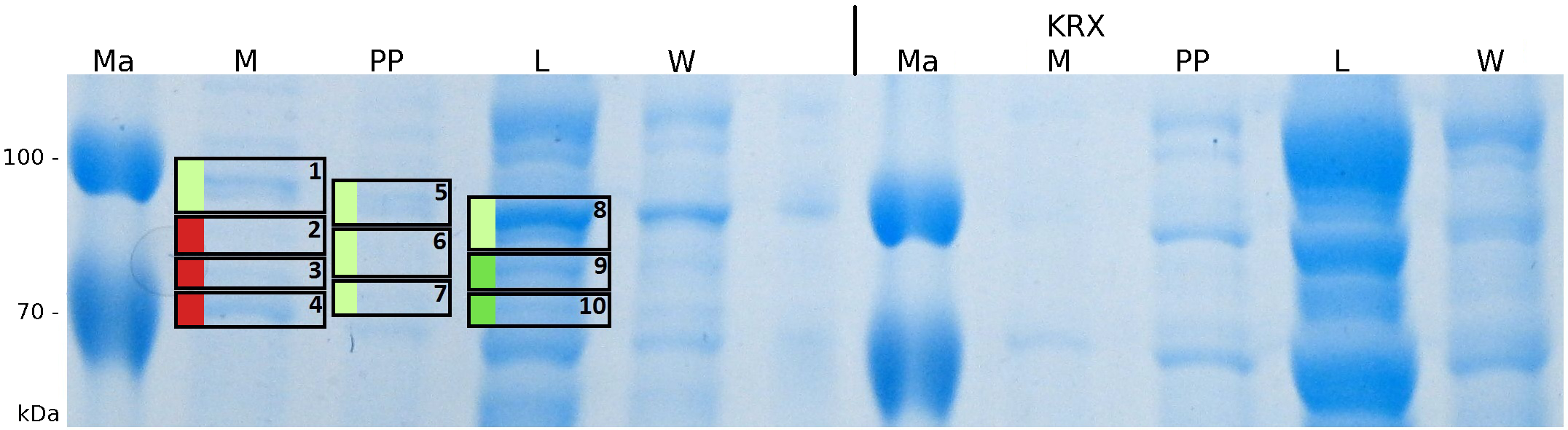
Sequence coverage could be identified in the supernatant of the media, in the periplasmatic fraction and in the cell lysis fraction, indicating that the TAT-sequence is working and that the protein is furthermore secreted into the media. This covers with the fluorescence measurements of the different fractions. A high fluorescence has been measured in the supernatant of the media, which is a remarkable characteristic because normally E. coli is only limited capable for secretion.
The influence of other detergents to disintegrate the S-layer fusion protein was tested after disrupting the cells with a ribolyser. The cell pellet was incubated in 10 % (v/v) Sodium dodecyl sulfate (SDS), in 7 M urea and 3 M thiourea (UTU), in 10 M urea (U) in 10 % (v/v) N-lauroyl sarcosine (NLS) and in 2 % CHAPS (C). Samples of the incubations with these detergents were loaded onto a SDS-PAGE prior to measurement with MALDI-TOF (see fig. 5).
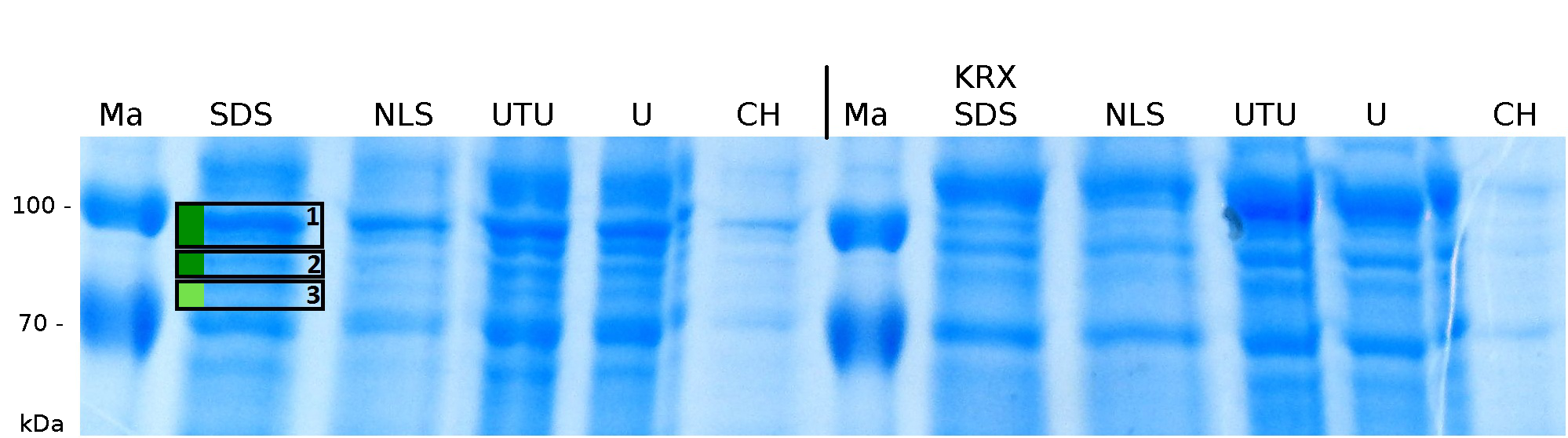
Altough most of the protein should be transported to the periplasm or secreted proteins should be found in the detergent fractions. So the SDS fraction was measured with MALDI-TOF, because SDS is able to solubilize nearly every protein. In the fractions of SDS sequence coverage over 20 % could be identified, indicating that a considerable amount of the fusion protein is located in the cytoplasm.
Purification
After the localisation of the S-layer protein in E. coli, different methods for purification were tested. The results of these methods are shown in fig. 6. Fig. 6 shows, that the CspB protein does not form inclusion bodies in E. coli and most of the protein is transported out of the cell into the periplasm and a lot of protein is even secreted into the medium (all fractions were concentrated by filtration and precipitation, respectively). The secretion into the culture medium is very interesting because the purification is much faster (no cell disruption necessary).
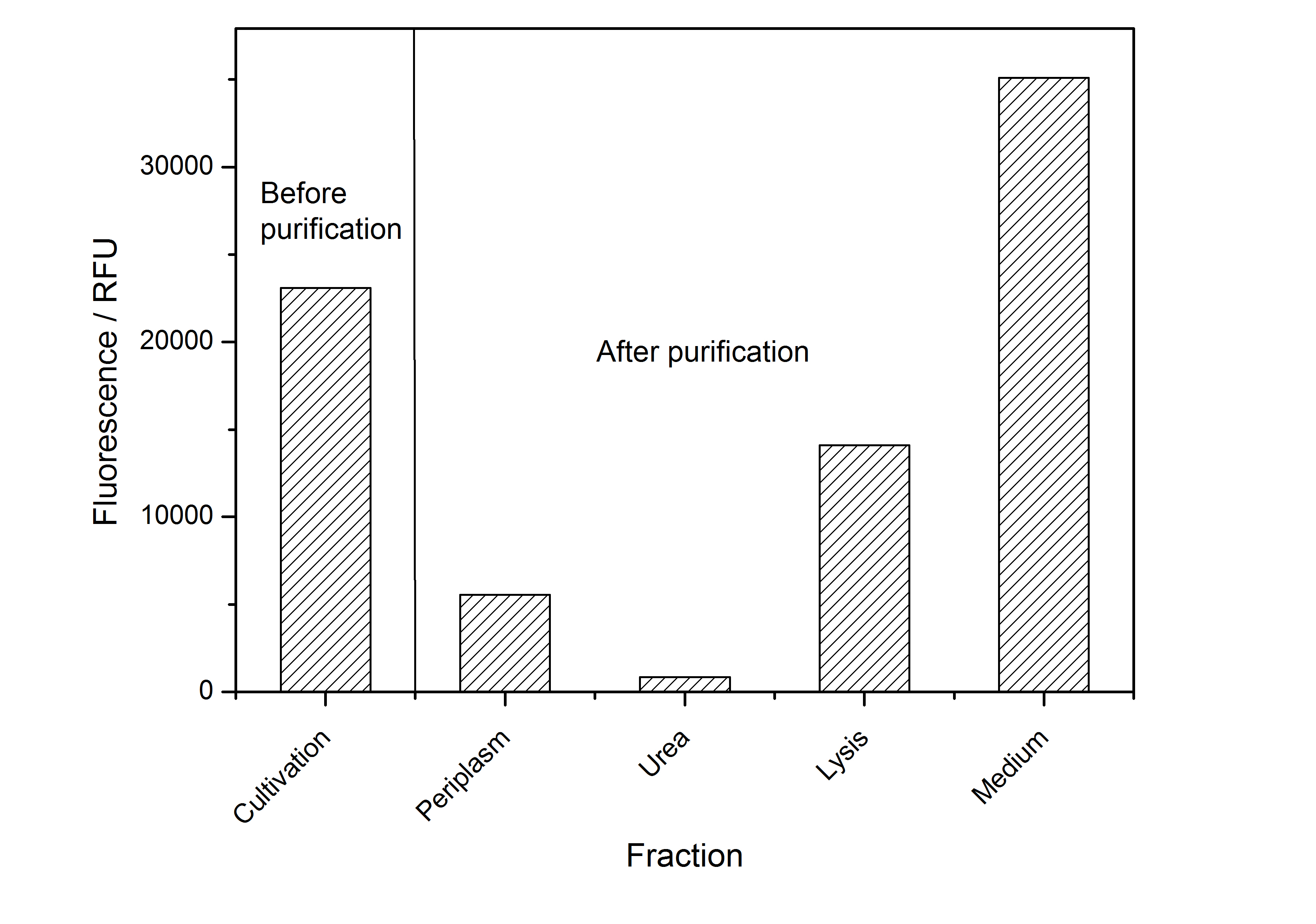
The highest fluorescence could be obtained by a precipitation with ammonium sulfate of the culture supernatant followed by an ultrafiltration with a 300 kDa membrane and a diafiltration with a 50 kDa membrane. The diafiltration was against a binding buffer for an anion exchange chromatography (25 mM sodium acetate, 25 mM sodium chloride) with pH 6, due to the theoretical pI of BBa_K525234. The fluorescence of the collected fractions of the following anion exchange chromatography are shown in fig. 7.
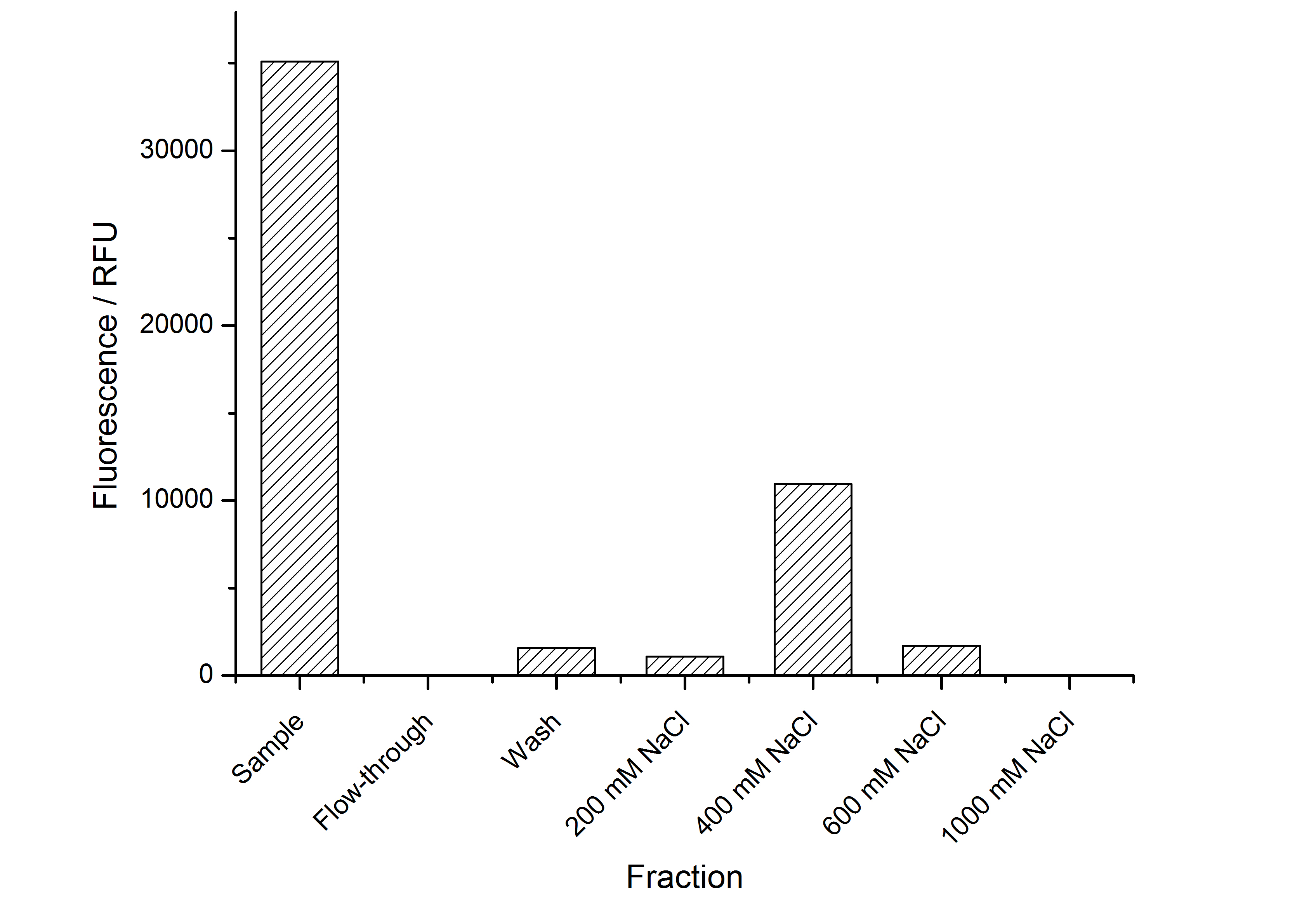
The binding conditions are well chosen because nearly all of the protein binds to the column. The protein is eluted from the column with rising sodium chloride concentrations. The highest fluorescence is in the elution fraction with 400 mM sodium chloride. 600 mM sodium chloride elutes all of the S-layer fusion proteins.
Methods
Expression of S-layer genes in E. coli
- Chassis: Promega's [http://www.promega.com/products/cloning-and-dna-markers/cloning-tools-and-competent-cells/bacterial-strains-and-competent-cells/single-step-_krx_-competent-cells/ E. coli KRX]
- Medium: LB medium supplemented with 20 mg L-1 chloramphenicol
- For autoinduction: Cultivations in LB-medium were supplemented with 0.1 % L-rhamnose as inducer and 0.05 % glucose
Measuring of mRFP
- Take at least 500 µL sample for each measurement (200 µL is needed for one measurement) so you can perform a repeat determination
- Freeze biological samples at -80 °C for storage, keep cell-free at 4 °C in the dark
- To measure the samples thaw at room temperature and fill 200 µL of each sample in one well of a black, flat bottom 96 well microtiter plate (perform at least a repeat determination)
- Measure the fluorescence in a platereader (we used a [http://www.tecan.com/platform/apps/product/index.asp?MenuID=1812&ID=1916&Menu=1&Item=21.2.10.1 Tecan Infinite® M200 platereader]) with following settings:
- 20 sec orbital shaking (1 mm amplitude with a frequency of 87.6 rpm)
- Measurement mode: Top
- Excitation: 584 nm
- Emission: 620 nm
- Number of reads: 25
- Manual gain: 100
- Integration time: 20 µs
Tryptic digest of gel lanes for analysis with MALDI-TOF
Note:
- Make sure to work under a fume hood.
- Do not work with protective gloves to prevent contamination of your sample with platicizers.
Reaction tubes have to be cleaned with 60% (v/v) CH3CN, 0.1% (v/v) TFA. Afterwards the solution has to be removed completely followed by evaporation of the tubes under a fume hood. Alternatively microtiter plates from Greiner® (REF 650161) can be used without washing.
- Cut out the protein lanes of a Coomassie-stained SDS-PAGE using a clean scalpel. Gel parts are transferred to the washed reaction tubes/microtiter plate. If necessary cut the parts to smaller slices.
- Gel slices should be washed two times. Therefore add 200 µL 30% (v/v) acetonitrile in 0.1 M ammonium hydrogen carbonate each time and shake lightly for 10 minutes. Remove supernatant and discard to special waste.
- Dry gel slices at least 30 minutes in a Speedvac.
- Rehydrate gel slices in 15 µL Trypsin-solution followed by short centrifugation.
- Gel slices have to be incubated 30 minutes at room temperature, followed by incubation at 37 °C over night.
- Dry gel slices at least 30 minutes in a Speedvac.
- According to the size of the gel slice, add 5 – 20 µL 50% (v/v) ACN / 0,1% (v/v) TFA.
- Samples can be used for MALDI measurement or stored at -20 °C.
Trypsin-solution: 1 µL Trypsin + 14 µL 10 mM NH4HCO3
- Therefore solubilize lyophilized Trypsin in 200 µL of provided buffer and incubate for 15 minutes at 30 °C for activation. For further use it can be stored at -20 °C.
Preparation and Spotting for analysis of peptides on Bruker AnchorChips
- Spot 0,5 – 1 µL sample aliquot
- Add 1 µL HCCA matrix solution to the spotted sample aliquots. Pipet up and down approximately five times to obtain a sufficient mixing. Be careful not to contact the AnchorChip.
Note: Most of the sample solvent needs to be gone in order to achieve a sufficiently low water content. When the matrix solution is added to the previously spotted sample aliquot at a too high water content in the mixture, it will result in undesired crystallization of the matrix outside the anchor spot area.
- Dry the prepared spots at room temperature
- Spot external calibrants on the adjacent calibrant spot positions. Use the calibrant stock solution (Bruker’s “Peptide Calibration Standard II”, Part number #222570), add 125 µL of 0,1 % TFA (v/v) in 30 % ACN to the vial. Vortex and sonicate the vial.
- Mix the calibrant stock solution in a 1:200 ratio with HCCA matrix and deposit 1 µL of the mixture onto the calibrant spots.
Complete protocol for production and purification of S-layer | mRFP fusion protein from C. halotolerans
- Cultivation
- 150 mL culture in 500 mL or 300 mL culture in 1000 mL shaking flasks with baffles (Schott) with silicon plugs
- Medium: [http://2011.igem.org/Team:Bielefeld-Germany/Protocols/Materials#Autoinduction_medium_for_KRX/ autoinduction medium]
- Cultivation temperature: 37 °C at 120 rpm
- Supernatant precipitation
- Centrifuge down the cells (10000 g, 30 min, 4 °C) and collect the supernatant
- To precipitate S-layer proteins from Corynebacterium, i.e. 40 % ammonium sulfate saturation concentration (247 g L-1 ammonium sulfate at 25 °C)
- Incubate 30 min at room temperature on a shaker
- Centrifuge (the faster and longer the better) and solve the precipitate in [http://2011.igem.org/Team:Bielefeld-Germany/Protocols/Materials#Materials#Buffers_for_S-layer_IEX/ binding buffer] for IEX (Ion exchange chromatography)

- Filtration
- Arrange the filtration module as shown on the right side.
- Collect permeate of cross flow filtration with 300 kDa membrane of sample before ultrafiltration
- This step is for removing cell debris
- Diafiltrate with 50 kDa membrane against [http://2011.igem.org/Team:Bielefeld-Germany/Protocols/Materials#Materials#Buffers_for_S-layer_IEX/ binding buffer] for IEX
- constantly delute permeate with the buffer, keeping the permeate volume as low as possible
- Used membranes: [http://www.millipore.com/catalogue/module/C7493 Milipore Pellicon XL 50], XL 100 or XL 300 membranes
- 50, 100 or 300 kDa cut-off
- 50 cm2 filtration area
- tangential flow filter
- Hydrophilic polyvinylidene fluoride membrane
- Used pump: SciLog TANDEM 1081 peristaltic pump
- flow rate during filtration: 40 mL min-1
- Ion exchange chromatography
- used column: DEAE HiTrap 1 mL with [http://www.gehealthcare.com/ GE Healthcare] ÄKTA
- flow rate: 1 mL min-1
- equilibrate column with 10 column volumes of [http://2011.igem.org/Team:Bielefeld-Germany/Protocols#Buffers_for_S-layer_IEX/ binding buffer]
- inject sample and wash column with binding buffer
- elute with 20 %, 40 % and 60 % [http://2011.igem.org/Team:Bielefeld-Germany/Protocols#Buffers_for_S-layer_IEX/ binding / elution buffer] mix and collect samples -> take the cleanest fraction for further work
- elute remaining proteins with 100 % elution buffer
References
Sleytr UB, Huber C, Ilk N, Pum D, Schuster B, Egelseer EM (2007) S-layers as a tool kit for nanobiotechnological applications, [http://onlinelibrary.wiley.com/doi/10.1111/j.1574-6968.2006.00573.x/full FEMS Microbiol Lett 267(2):131-144].
//proteindomain/internal
//rnap/bacteriophage/t7
None |