
Part:BBa_K3900028
RUBY Plant Reporter
RUBY Plant Reporter
Contents
Usage and Biology
RUBY is a reporter system for plants that changes the plants’ color to red since it is based on the plant pigments betalains [1,2]. Betalains belong to one of the major pigment classes in plants, are water-soluble and have a strong antioxidant activity [3]. They can be divided into two groups, the yellow betaxanthins and the red-violet betacyanins with absorption maxima at 460-480 nm and 535-538 nm, respectively [4]. Betalain, the final product of the RUBY reporter, belongs to the betacyanins.
Although the betalain biosynthesis is naturally based on a complex network of enzymes and intermediates, RUBY uses only three enzymes to convert tyrosine into betalain: a cytochrome P450 (encoded by CYP76AD1), DODA and a glycosyl transferase [4]. These enzymes are coded in a single open reading frame. They are separated by 2A peptides, enabling self-cleavage of the fusion protein to obtain three individual and functional enzymes (see figure 1).
To this date, several different kinds of reporter systems are used that have disadvantages when used for a biosensor in comparison to the RUBY reporter. Degreening reporter inferfere with the photosynthesis, thereby limit the photosynthetic activity and the survival of the plant. Other reporters like luciferase are in need of an additional substrate before they are visible. RUBY however is visible by the naked eye and only requires tyrosine as a substrate. RUBY has a broad range of applications: it can be used as a reporter for cell signaling but also in a biosensor. It has not been used in iGEM so far and is therefore a great addition for all plant projects.
Transient transformation of RUBY
To assess the properties of the RUBY reporter, we transiently transformed RUBY under control of the constitutive CaMV 35S promoter in leaves of N. benthamiana plants. Upon transformation with 35S:RUBY, the leaf color changed clearly visible to red during the following days (see figure 2). Even at the day of WikiFreeze (21st October 2021), the leaves were still showing a vibrant red coloration, confirming that the RUBY pigments stay stable in plant leaves for a long time (Fig. 3). Several kinds of analyses of this color change were performed, which are presented in the following paragraphs.
Betalain extraction and quantification
Betalains are the final products of the RUBY reporter. To investigate how the betalain content of transformed leaves changes over time, we extracted and quantified betalain 1 day, 2 days, 3 days, 7 days, 8 weeks and 9 weeks after transformation. For extraction, 1 cm² of the leaf was cut out and weighed. A small amount of extraction buffer (35 % EtOH, pH 5) was added to the sample, which was then lysed with a ribolyzer (Precellys 24). After the lyses, the volume of the extraction buffer was added up to 1 mL. After centrifugation, the supernatant was transferred in cuvettes and the absorption at 535 nm was measured in a spectrophotometer. In the end, the betalain content in mg per g fresh weight was calculated with the formula [5] in Figure 4.
The analysis (Fig. 5) shows that at the first day after transformation, the betalain content was significantly lower than at all the other time points. At every following time point, the betalain content was high, with a slight decrease from 8 weeks to 9 weeks. This shows that after the transformation of RUBY under the control of the constitutive CaMV 35S promoter, the pigments produced by the RUBY reporter are present in the leaves for a very long time.
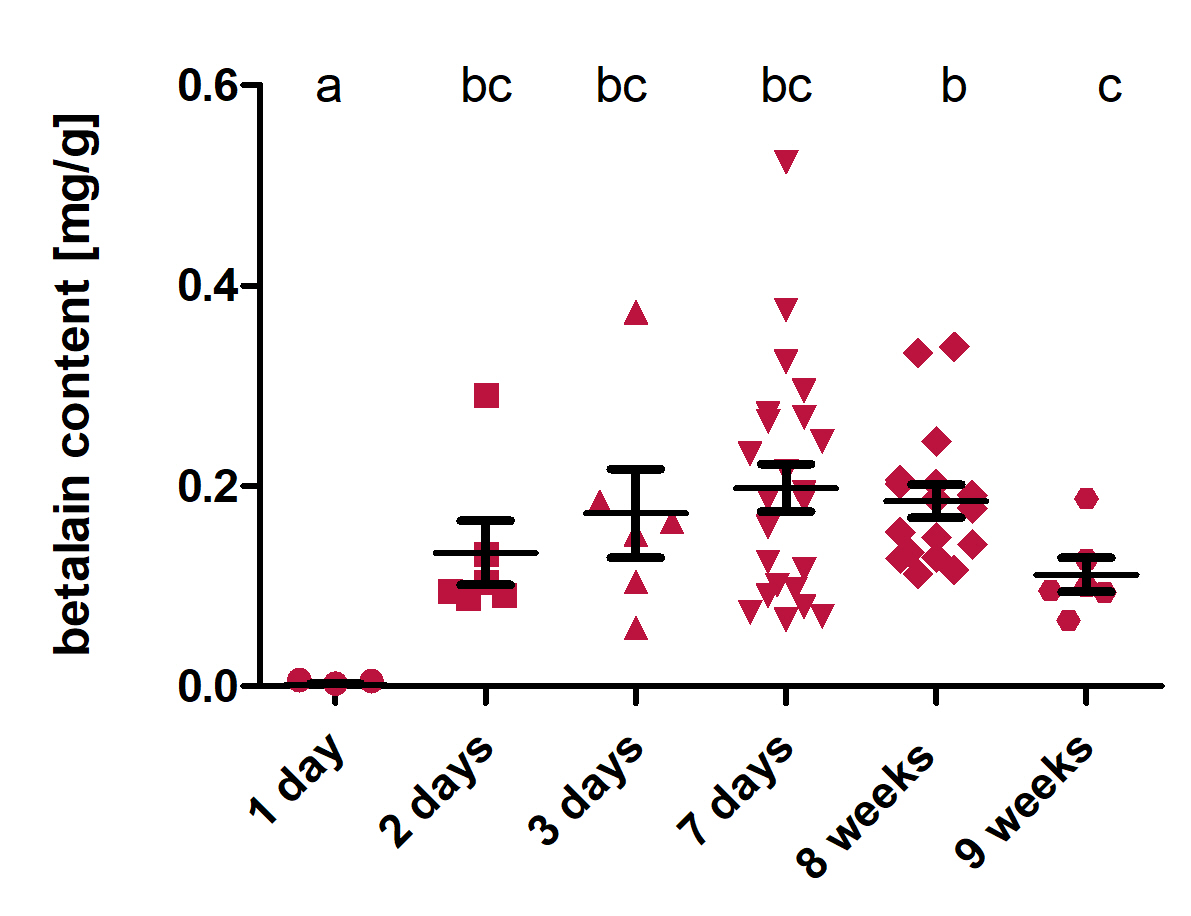
Transcript Analysis
To ensure that all three RUBY enzymes were expressed, we performed a transcript analysis at two different time points after agroinfiltration to analyze the relative expression of CYP76AD1α, DODAα and glycosyl transferase. The time points day two and day seven were chosen, because transient expression with agroinfiltration is highest after two days and should decrease over time [6].
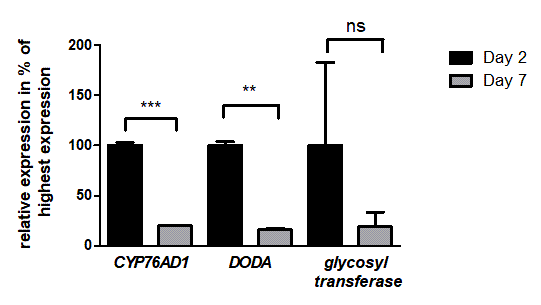
The results show that all three genes responsible for the betalain production are in fact expressed after two and seven days. Furthermore, we were also able to show that the expression is significantly higher at two days in comparison to seven days for the genes CYP76AD1&alpha and DODA&alpha (Fig. 6).
PAM measurement for analysis of photosynthesis
The measurement of chlorophyll fluorescence is a powerful tool to investigate and elucidate the complex regulation of photosynthesis. This is due to the tight connection of the three possible reactions of de-excitation of the chlorophylls in photosystem II (PSII): photochemical reactions, emission of fluorescence and emission of heat. The portable chlorophyll fluorometer “MINI-PAM”, built by the Heinrich Walz GmbH, is a great device to record and evaluate different characteristics of chlorophyll fluorescence. The most prominent are the yield of photosystem II and the non-photochemical quenching (NPQ), the process of enhanced conversion of absorbed light energy for harmlessly dissipating excess excitation energy. The PAM measurement is a very complex process. But in the end, the information is assessed by two consecutive measures of fluorescence yield, one briefly before and one during a short pulse of saturating light.
To assess the influence of the expression of the RUBY reporter on the efficiency of photosynthesis, we performed two different experiments with the MINI-PAM to measure three different parameters. As first experiment we measured the PSII yield at ambient light conditions of infiltrated and control areas of each two leaves of in total twelve plants, with three replicates of each measurement. In the second experiment, we measured the light curve of a dark adapted leaf with five replicates for the infiltrated area and the control area. With this light curve, it was possible to measure both PSII yield and NPQ depending on the light intensity.
The measurement of PSII yield at ambient light conditions in the first experiment shows that the leaves in both conditions, expressing RUBY and control, both show the same PSII yield of a bit less than 80 % (see figure 7). This indicates that the expression of the RUBY reporter does not impair the photosynthesis at normal light conditions.
The light curve experiment shows that with increasing light intensity the PSII yield of the leaves expressing the RUBY reporter is slightly decreased compared to not transformed areas of the leaves (see figure 8). Apparently, the expression of the RUBY reporter impairs the ability of the leaf to cope with high light intensities. At the same time, the expression of RUBY leads to increased NPQ with increasing light intensity (see figure 9).
Estrogen induced expression of RUBY in Nicotiana benthamiana
In order to determine if RUBY is not only expressible with a constitutive promoter, but also with an inducible one, we used an estrogen-based induction system. There, RUBY was put under the control of the lexA promoter. A slight red coloration of the leaves was visible (Fig. 10). Consequently, RUBY is suitable as an inducible reporter in plants. This can also be confirmed by recent research of Alfonso Timoneda et al., who used betalain pigments to visualize arbuscular mycorrhizal colonisation [8].
Metabolome
In order to determine if, how much and where the expression of the RUBY reporter impacts the metabolome of Nicotiana benthamiana, a gas chromatography coupled with mass spectroscopy (GC/MS) analysis was performed. This is of interest, as the biosynthesis that is used to produce visible red betalains uses tyrosine as a precursor [1]. However, tyrosine is also a precursor of several important products involved in defense, pollinator attraction, electron transport and structural support [9]. In addition, tyrosine shares precursors with other aromatic amino acids [8]. The increased use of these substances could cause downstream effects. Therefore, the goal is to determine whether other pathways are disturbed by changes in tyrosine levels, as this would need to be taken into account, when using RUBY as a reporter.
Sampling
Different samples were used for the analysis of the metabolome:
1. The part of the leaf, that was infiltrated with agrobacteria containing RUBY (“R”)
2. The same leaf, but at a site that was not infiltrated (“G”)
3. Another leaf of the same plant (“K”)
The G-samples serve as a comparison for the R-samples. As changes that affect some part of the leaf could also affect the whole leaf, other leaves that were not infiltrated were also taken into account. In theory, changes could even affect the entire plant. However, sampling additional plants that were not infiltrated at all would have made it difficult to distinguish, whether changes are caused by RUBY expression, or due to differences in development, light conditions or further influences.
Samples were taken from five plants, each with three similar old leaves infiltrated. Patches from each plant were combined to form a replicate. The R-sample of a plant therefore consists of the red leaf tissue of all three infiltrated leaves. The G-sample consists of the green tissue samples of these leaves and the K-samples of tissue samples from 2 other leaves. The advantage of combining several leaves is that variance due to differences in development between leaves that differ in age, should be averaged out.
Betalains
First, the presence of betanin was analyzed for the samples, as without its presence, we would not expect any changes. Betanin is one of the major components produced by RUBY [4]. Its abundance was detected using liquid chromatography combined with mass spectroscopy (LC/MS). In figure 11 it can be seen that for all five R-samples there is a clear detection peak for betanin, while it is not visible for the G-samples. That confirms that betanin was synthesized in the infiltrated tissue, but not in the rest of the leaf. This also verifies that betanin does not spread inside the leaf, which could otherwise impact the control samples.
Tyrosine
Further metabolites were analyzed using GC/MS, as this allows a more complete overview of the metabolome than LC. As a first step, the tyrosine levels were analyzed, as most of the changes will be connected to changes in tyrosine levels. To assure a correct identification, a tandem mass spectroscopy measurement was performed. As expected, tyrosine levels change significantly with a significance level of 0.01 (figure 12).
Metabolome
In total, 94 metabolites could be identified in the samples. Peak areas were normalized with an internal standard as well as the initial weight of the sample. A principal component analysis (PCA) was performed, to check whether the Ruby infiltration is the major cause of variance between samples. The plot of the first two principal components (PC) is shown in figure 13.
As it can be seen, differently treated samples separated in clusters along the first PC. This is especially true for the R-samples that clearly separate from all other samples. This indicates that the infiltration indeed is the major cause of variance between samples. The G- and R-samples lie closer together than the K- and R-samples, which supports the hypothesis that changes caused by RUBY affect the entire leaf and not only the infiltrated patch. In the second PC, the variance inside the treatment groups becomes more important than the difference between groups. There is one outlier (sample K1) which is similar to one of the G-samples. A possibility is to exclude this sample from further analyses. This was not done because it is not clear what the reason for this outlier might be. The first PC explains 39% of the variance, the second PC another 19%. This suggests having a look at the third PC, which adds another 9%. The resulting three dimensional plot is shown in figure 14. However, this only shows some additional variance inside of treatment groups and therefore is not relevant for assessment of changes due to infiltration.
As a next step, statistical analysis of the detection for each metabolite was performed. Results are shown as volcano-plots. For the comparison between R- and G-samples (figure 15), it can be seen that indeed several metabolites changed significantly (significance level of 0.01 (red dots)). Except for Glycerol-3-P, all significantly changed metabolites increased due to RUBY expression.

This increase was even higher than change in tyrosine levels, for which a decrease in abundance was detected. When the biosynthesis of tyrosine is further examined, it stands out that several precursors are changed, starting from glycolysis (Figure 16) with glucose-6-phosphate, fructose-6-phosphate and pyruvate. The glycolysis is connected to tyrosine and further aromatic amino acids through the shikimate-pathway of which shikimate has increased (Figure 17). This supports the hypothesis that the reactions leading to tyrosine are upregulated. The decrease of tyrosine is likely due to conversion into betalains. As we could still detect , it is likely that the increase of precursors allows most of the additional required tyrosine to be reproduced. Another amino acid of the same biosynthesis pathway that is affected by the changes is tryptophan. Its abundance is increased but also with smaller significance than the precursors. This may be a side effect of their upregulation.
However, there are other influenced metabolites such as sucrose and cellobiose. This can be another side-effect of an increased sugar metabolism. Further explanations can be the need of sugars to form betalain from betanidin or repair of damage to cell walls or membranes due to infiltration.
Comparison of G to K leads to no significant changes. That means that the effect of RUBY expression does not affect the rest of the leaf as much as the infiltrated spot.
In conclusion, we could show that the RUBY expression influences the metabolome, which can be traced back to be connected with the higher demand of the betalain precursor tyrosine. Tyrosine itself can be reproduced fast enough so that the cell does not run out of it. It should be taken into account when using RUBY as a reporter, the metabolome is influenced and therefore can influence the results.
References
[1] He, Y., Zhang, T., Sun, H. et al. A reporter for noninvasively monitoring gene expression and plant transformation. Hortic Res 7, 152 (2020).
[2] Tanaka, Y., Sasaki, N. & Ohmiya, A. Biosynthesis of plant pigments: anthocyanins, betalains and carotenoids. Plant J 54, 733–749; (2008).
[3] Escribano, J., Pedreño, M. A., García-Carmona, F. & Muñoz, R. Characterization of the antiradical activity of betalains from Beta vulgaris L. roots. Phytochem. Anal. 9, 124–127; (1998).
[4] Khan, M. I. & Giridhar, P. Plant betalains: Chemistry and biochemistry. Phytochemistry 117, 267–295; (2015)
[5] Kumar, S. S., Manoj, P., Shetty, N. P., Prakash, M. & Giridhar, P. Characterization of major betalain pigments -gomphrenin, betanin and isobetanin from Basella rubra L. fruit and evaluation of efficacy as a natural colourant in product (ice cream) development. J Food Sci Technol 52, 4994–5002 (2015).
[6] Ma, L., Lukasik, E., Gawehns, F. & Takken, F. L. W. The Use of Agroinfiltration for Transient Expression of Plant Resistance and Fungal Effector Proteins in Nicotiana benthamiana Leaves. in Plant Fungal Pathogens: Methods and Protocols (eds. Bolton, M. D. & Thomma, B. P. H. J.) 61–74 (2012).
[7] PHOTOSYNTHESIS YIELD ANALYZER MINI-PAM. Portable Chlorophyll Fluorometer. Handbook of Operation. https://www.walz.com/products/chl_p700/mini-pam-II/downloads.html
[8]Timoneda, A. et al. MycoRed: Betalain pigments enable in vivo real-time visualisation of arbuscular mycorrhizal colonisation. PLoS Biol 19, e3001326; 10.1371/journal.pbio.3001326 (2021).
[9] Schenck, Craig A., et al. Tyrosine biosynthesis, metabolism, and catabolism in plants. Phytochemistry 149: 82-102. (2018)
[10]Kanehisa, Minoru. The KEGG database. Novartis found symp. Vol. 247. (2002)
None |