Difference between revisions of "Part:BBa K801043"
(→Contribution of OUC-China) |
|||
(7 intermediate revisions by 2 users not shown) | |||
Line 3: | Line 3: | ||
composite part of Bba_K319003, K801039, BBa_K801011 , Bba_K319003, Bba_K801041, and BBa_K801011 | composite part of Bba_K319003, K801039, BBa_K801011 , Bba_K319003, Bba_K801041, and BBa_K801011 | ||
+ | |||
+ | =Contribution of OUC-China= | ||
+ | '''Group''': [https://2023.igem.wiki/ouc-china/parts iGEM Team OUC-China 2023] | ||
+ | |||
+ | '''Author''': Qingyu Wu | ||
+ | |||
+ | '''Summary''': Red light optimization | ||
+ | |||
+ | Due to the fact that the fermentation of MEL-producing sap is thicker, it may cause the red light system to not work well. We therefore used a simulation based on Rombauer's law to model the propagation of light through the sap during fermentation to determine what lighting arrangement scheme we could use to maximize fermentation with minimal energy consumption in order to reduce the expense of subsequent experiments and increase their success. | ||
+ | <html> | ||
+ | |||
+ | <figure> | ||
+ | |||
+ | <img src="https://static.igem.wiki/teams/4711/wiki/model/12-red-light-system-arrangement-chat.png"width="100%" style="float:center"> | ||
+ | |||
+ | <figcaption> | ||
+ | |||
+ | <p style="font-size:1rem"> | ||
+ | |||
+ | |||
+ | |||
+ | |||
+ | </figcaption> | ||
+ | |||
+ | </figure> | ||
+ | </html> | ||
+ | |||
+ | |||
+ | I = I0 * e^(-αx) | ||
+ | |||
+ | |||
+ | <html> | ||
+ | |||
+ | <figure> | ||
+ | |||
+ | <img src="https://static.igem.wiki/teams/4711/wiki/222.png"width="100%" style="float:center"> | ||
+ | |||
+ | <figcaption> | ||
+ | |||
+ | <p style="font-size:1rem"> | ||
+ | |||
+ | |||
+ | |||
+ | |||
+ | </figcaption> | ||
+ | |||
+ | </figure> | ||
+ | </html> | ||
+ | |||
+ | The fermentation tank we used is a cylindrical vessel with a radius of approximately 10cm and a height of approximately 40cm. Considering the actual fermentation process, most of the fermentation culture is located in the upper layer, while the lower layer is mainly occupied by the product MEL. After continuous adjustment of the number and positioning of the lights, we finally determined that wrapping 8 light bulbs with an intensity of 180 umol/m^2/nm at distances of 4cm, 12cm, and 20cm from the top of the tank would ensure that the upper 2/3 region within the fermentation tank reaches a light intensity of 50 umol/m^2/nm. | ||
+ | |||
+ | |||
==Background and principles== | ==Background and principles== | ||
Line 30: | Line 82: | ||
[[Image:TUM12 modelling PCB binding cavity PhyB.jpg|thumb|left|400px|Cavity of PCB binding pocket of PhyB, predicted by I-TASSER. The next most homolog protein is illustrated in cyan, the cyanobacterial phytochrome CPH1 [http://www.rcsb.org/pdb/explore.do?structureId=2VEA 2VEA]. The golden ribbon indicates the predicted structure of PhyB. The sulfhydryl group of the ''Arabidopsis'' chromophore-binding cysteine residue is co-ordinated with the position of the ethylidene moiety on the chromophore sufficiently closely and in the correct conformation to form the thioether bond by which the chromophore is known to be covalently attached.]] | [[Image:TUM12 modelling PCB binding cavity PhyB.jpg|thumb|left|400px|Cavity of PCB binding pocket of PhyB, predicted by I-TASSER. The next most homolog protein is illustrated in cyan, the cyanobacterial phytochrome CPH1 [http://www.rcsb.org/pdb/explore.do?structureId=2VEA 2VEA]. The golden ribbon indicates the predicted structure of PhyB. The sulfhydryl group of the ''Arabidopsis'' chromophore-binding cysteine residue is co-ordinated with the position of the ethylidene moiety on the chromophore sufficiently closely and in the correct conformation to form the thioether bond by which the chromophore is known to be covalently attached.]] | ||
+ | <br/><br/><br/><br/><br/><br/><br/><br/><br/><br/><br/><br/><br/><br/><br/><br/><br/><br/><br/> | ||
+ | |||
+ | =References= | ||
+ | *[[http://www.ncbi.nlm.nih.gov/pubmed/15823535 Chen et al., 2005]] Chen, M., Tao, Y., Lim, J., Shaw, A., and Chory, J. (2005). Regulation of phytochrome B nuclear localization through light-dependent unmasking of nuclear-localization signals. ''Curr Biol'', 15(7):637–42. | ||
+ | *[[http://www.ncbi.nlm.nih.gov/pubmed/19165330 Kikis et al., 2009]] Kikis, E. A., Oka, Y., Hudson, M. E., Nagatani, A., and Quail, P. H. (2009). Residues clustered in the light-sensing knot of phytochrome B are necessary for conformer-specific binding to signaling partner PIF3. ''PLoS Genet'', 5(1):e1000352. | ||
+ | *[[http://www.ncbi.nlm.nih.gov/pubmed/19749742 Levskaya et al., 2009]] Levskaya, A., Weiner, O. D., Lim, W. A., and Voigt, C. A. (2009). Spatiotemporal control of cell signalling using a light-switchable protein interaction. ''Nature'', 461(7266):997–1001. | ||
+ | *[[http://www.ncbi.nlm.nih.gov/pubmed/12355112 Mendelsohn, 2002]] Mendelsohn, A. R. (2002). An enlightened genetic switch. ''Nat Biotechnol'', 20(10):985–7. | ||
+ | *[[http://www.ncbi.nlm.nih.gov/pubmed/12219076 Shimizu-Sato et al., 2002]] Shimizu-Sato, S., Huq, E., Tepperman, J. M., and Quail, P. H. (2002). A light-switchable gene promoter system. ''Nat Biotechnol'', 20(10):1041–4. | ||
+ | *[[http://www.ncbi.nlm.nih.gov/pubmed/15486100 Khanna et al., 2004]] Khanna, R., Huq, E., Kikis, E. A., Al-Sady, B., Lanzatella, C., and Quail, P. H. (2004). A novel molecular recognition motif necessary for targeting photoactivated phytochrome signaling to specific basic helix-loop-helix transcription factors. ''Plant Cell'', 16(11):3033–44. | ||
+ | *[[http://www.ncbi.nlm.nih.gov/pubmed/11553807 Gambetta and Lagarias, 2001]] Gambetta, G. A. and Lagarias, J. C. (2001). Genetic engineering of phytochrome biosynthesis in bacteria. ''Proc Natl Acad Sci U S A'', 98(19):10566–71. | ||
+ | *[[http://www.ncbi.nlm.nih.gov/pubmed/10466729 Ni et al., 1999]] Ni, M., Tepperman, J. M., and Quail, P. H. (1999). Binding of phytochrome B to its nuclear signalling partner PIF3 is reversibly induced by light. ''Nature'', 400(6746):781–4. | ||
+ | *[[http://www.ncbi.nlm.nih.gov/pubmed/12734586 Van Criekinge and Beyaert, 1999]] Van Criekinge, W. and Beyaert, R. (1999). Yeast two-hybrid: State of the art. ''Biol Proced Online'', 2:1–38. | ||
+ | *[[http://www.ncbi.nlm.nih.gov/pubmed/3891738 Wertman and Mount, 1985]] Wertman, K. F. and Mount, D. W. (1985). Nucleotide sequence binding specificity of the LexA repressor of ''Escherichia coli'' K-12. ''J Bacteriol'', 163(1):376–84. | ||
<!-- Add more about the biology of this part here | <!-- Add more about the biology of this part here |
Latest revision as of 12:29, 12 October 2023
LexA based yeast light-swithable promoter system
composite part of Bba_K319003, K801039, BBa_K801011 , Bba_K319003, Bba_K801041, and BBa_K801011
Contribution of OUC-China
Group: iGEM Team OUC-China 2023
Author: Qingyu Wu
Summary: Red light optimization
Due to the fact that the fermentation of MEL-producing sap is thicker, it may cause the red light system to not work well. We therefore used a simulation based on Rombauer's law to model the propagation of light through the sap during fermentation to determine what lighting arrangement scheme we could use to maximize fermentation with minimal energy consumption in order to reduce the expense of subsequent experiments and increase their success.
I = I0 * e^(-αx)
The fermentation tank we used is a cylindrical vessel with a radius of approximately 10cm and a height of approximately 40cm. Considering the actual fermentation process, most of the fermentation culture is located in the upper layer, while the lower layer is mainly occupied by the product MEL. After continuous adjustment of the number and positioning of the lights, we finally determined that wrapping 8 light bulbs with an intensity of 180 umol/m^2/nm at distances of 4cm, 12cm, and 20cm from the top of the tank would ensure that the upper 2/3 region within the fermentation tank reaches a light intensity of 50 umol/m^2/nm.
Background and principles
This system bases on the yeast two-hybrid system which was originally created for exploring protein-protein interactions. One candidate of a potential protein-interaction pair is fused to the DNA-binding domain of a transcription factor and the other candidate to the activation domain of a transcription factor. If the proteins candidates are really physically interacting with each other, this event will starts the transcription of downstream reporter genes, e. g. LacZ or an auxotrophic marker.
Reverse yeast-two hybrid based light-switchable promoter system
This basic principle is utilized in the yeast light-switchable promoter system. But in contrast to yeast-two hybrid, we already know the interaction partners (PhyB and PIF3). The photoconvertible binding of PhyB to PIF3 is used, to recover the physical contiguity of the DNA binding domain and the transcriptional activation domain under defined conditions (red light).
This light-inducible system contains two proteins, phytochrome B (PhyB) and phytochrome interacting factor 3 (PIF3). PhyB and PIF3 will just form a heterodimer, if PhyB is exposed to red light. Exposition under red light leads to a conformation change of PhyB to its active form (Pfr-form); the Pfr form of PhyB now can bind PIF3. PhyB comprises a light-absorbing chromophore phycocyanobilin, which gives PhyB the ability to undergo a photoconversion to the active Pfr form (red light exposition) or back to its ground-state Pr (far-red light exposition or darkness).
GAL4 based light-switchable promoter system
For more information about the GAL4 based system, please see here: BBa_K801042
LexA based light-switchable-promoter system
In contrast to the GAL4 based light-switchable promoter system there is no need for KO of GAL4/GAL80 genes in yeast with a LexA based light-switchable promoter system. The difference is that we use LexA, a prokaryotic DNA binding protein, for the DNA binding part of our light-switchable promoter system, instead of GAL4DBD. LexA does not interfere with the endogenous yeast metabolism and signalling system because it only recognizes a special prokaryotic DNA sequence, the so-called LexA operator (=LexA binding site). LexA binding sites can be used upstream of a minimal promoter (=TATA box) to be utilized as a cis-acting regulatory element.
In this case the genes, which we want to control by light, have to be cloned downstream of a synthetic promoter containing a minimal promoter, preceded by multiple LexA binding sites, e. g. BBa_K165031.
In distinction from the GAL4 based system there is no necessity for a special strain carrying an GAL4/80 deletion, so theoretically every yeast strain can be used for this system.
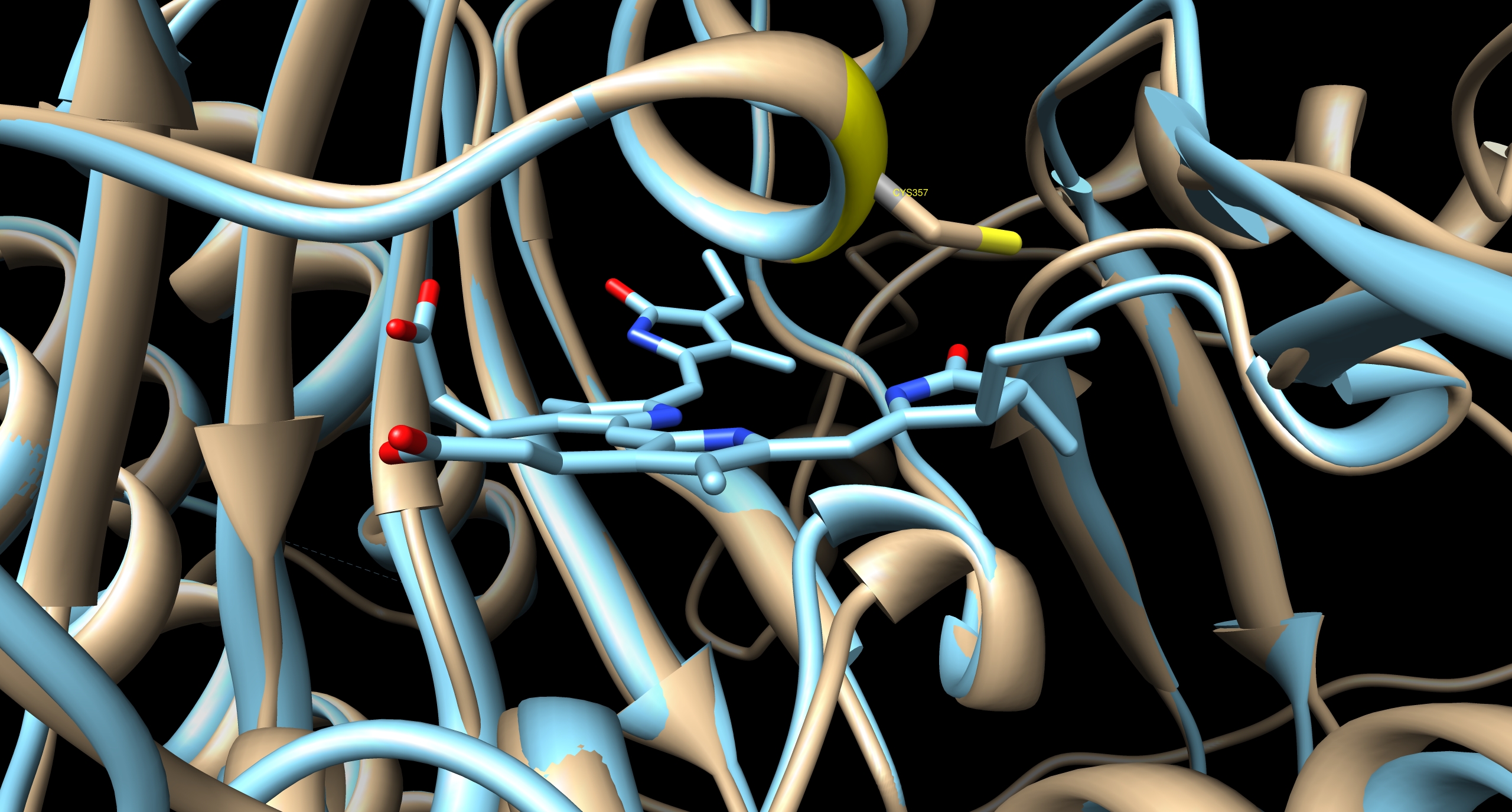
References
- http://www.ncbi.nlm.nih.gov/pubmed/15823535 Chen et al., 2005 Chen, M., Tao, Y., Lim, J., Shaw, A., and Chory, J. (2005). Regulation of phytochrome B nuclear localization through light-dependent unmasking of nuclear-localization signals. Curr Biol, 15(7):637–42.
- http://www.ncbi.nlm.nih.gov/pubmed/19165330 Kikis et al., 2009 Kikis, E. A., Oka, Y., Hudson, M. E., Nagatani, A., and Quail, P. H. (2009). Residues clustered in the light-sensing knot of phytochrome B are necessary for conformer-specific binding to signaling partner PIF3. PLoS Genet, 5(1):e1000352.
- http://www.ncbi.nlm.nih.gov/pubmed/19749742 Levskaya et al., 2009 Levskaya, A., Weiner, O. D., Lim, W. A., and Voigt, C. A. (2009). Spatiotemporal control of cell signalling using a light-switchable protein interaction. Nature, 461(7266):997–1001.
- http://www.ncbi.nlm.nih.gov/pubmed/12355112 Mendelsohn, 2002 Mendelsohn, A. R. (2002). An enlightened genetic switch. Nat Biotechnol, 20(10):985–7.
- http://www.ncbi.nlm.nih.gov/pubmed/12219076 Shimizu-Sato et al., 2002 Shimizu-Sato, S., Huq, E., Tepperman, J. M., and Quail, P. H. (2002). A light-switchable gene promoter system. Nat Biotechnol, 20(10):1041–4.
- http://www.ncbi.nlm.nih.gov/pubmed/15486100 Khanna et al., 2004 Khanna, R., Huq, E., Kikis, E. A., Al-Sady, B., Lanzatella, C., and Quail, P. H. (2004). A novel molecular recognition motif necessary for targeting photoactivated phytochrome signaling to specific basic helix-loop-helix transcription factors. Plant Cell, 16(11):3033–44.
- http://www.ncbi.nlm.nih.gov/pubmed/11553807 Gambetta and Lagarias, 2001 Gambetta, G. A. and Lagarias, J. C. (2001). Genetic engineering of phytochrome biosynthesis in bacteria. Proc Natl Acad Sci U S A, 98(19):10566–71.
- http://www.ncbi.nlm.nih.gov/pubmed/10466729 Ni et al., 1999 Ni, M., Tepperman, J. M., and Quail, P. H. (1999). Binding of phytochrome B to its nuclear signalling partner PIF3 is reversibly induced by light. Nature, 400(6746):781–4.
- http://www.ncbi.nlm.nih.gov/pubmed/12734586 Van Criekinge and Beyaert, 1999 Van Criekinge, W. and Beyaert, R. (1999). Yeast two-hybrid: State of the art. Biol Proced Online, 2:1–38.
- http://www.ncbi.nlm.nih.gov/pubmed/3891738 Wertman and Mount, 1985 Wertman, K. F. and Mount, D. W. (1985). Nucleotide sequence binding specificity of the LexA repressor of Escherichia coli K-12. J Bacteriol, 163(1):376–84.
Sequence and Features
- 10COMPATIBLE WITH RFC[10]
- 12COMPATIBLE WITH RFC[12]
- 21INCOMPATIBLE WITH RFC[21]Illegal BglII site found at 862
Illegal BglII site found at 2795
Illegal BglII site found at 5635
Illegal BamHI site found at 2877
Illegal XhoI site found at 2828
Illegal XhoI site found at 2847
Illegal XhoI site found at 4982 - 23COMPATIBLE WITH RFC[23]
- 25INCOMPATIBLE WITH RFC[25]Illegal NgoMIV site found at 2240
Illegal AgeI site found at 5705 - 1000INCOMPATIBLE WITH RFC[1000]Illegal BsaI site found at 4367
Illegal BsaI site found at 4773
Illegal BsaI.rc site found at 205
Illegal BsaI.rc site found at 1986
Illegal SapI site found at 3044