Difference between revisions of "Part:BBa K1330000"
Neha adarsh (Talk | contribs) |
Neha adarsh (Talk | contribs) |
||
Line 64: | Line 64: | ||
<p>Using these sequences we ran GROMACS simulation to find the energy minimization results. These results were plotted using the steepest descent algorithm and it was observed that the native structure converged in almost 200 steps (Figure 4a) while for Spinach2.6 it converged in nearly 180 steps (Figure 4d). An NVT equilibration was performed and trajectory files were generated to visualise the simulation. It was found that the system reached equilibrium at less than 20 ps (Figure 4b, e). This equilibrated system was subjected to the Steer MD simulations to dislocate the ligand from the G-Quadruplex binding site with spring force 10000 KJ/mol/nm2, see the Figure 4c, d. The force applied was able to pull the ligand apart from the binding site of the RNA aptamer as seen in the animation below. Overall, the energy minimization plot suggests that the Spinach2.6 complex structure is relatively stable when compared with the native structure. Moreover, the Steer MD plots for both these complexes are significantly different suggesting the binding affinities of the ligand to RNA aptamer structure are different.</p><br> | <p>Using these sequences we ran GROMACS simulation to find the energy minimization results. These results were plotted using the steepest descent algorithm and it was observed that the native structure converged in almost 200 steps (Figure 4a) while for Spinach2.6 it converged in nearly 180 steps (Figure 4d). An NVT equilibration was performed and trajectory files were generated to visualise the simulation. It was found that the system reached equilibrium at less than 20 ps (Figure 4b, e). This equilibrated system was subjected to the Steer MD simulations to dislocate the ligand from the G-Quadruplex binding site with spring force 10000 KJ/mol/nm2, see the Figure 4c, d. The force applied was able to pull the ligand apart from the binding site of the RNA aptamer as seen in the animation below. Overall, the energy minimization plot suggests that the Spinach2.6 complex structure is relatively stable when compared with the native structure. Moreover, the Steer MD plots for both these complexes are significantly different suggesting the binding affinities of the ligand to RNA aptamer structure are different.</p><br> | ||
− | [[File:Parts-mod-Figure4_( | + | [[File:Parts-mod-Figure4_(2).jpg]] |
Revision as of 01:07, 14 October 2022
Spinach2.1 flanked by tRNALys3
This BioBrick contains the gene coding for the Spinach2.1 RNA, flanked by a tRNA scaffold sequence.
Usage and Biology
Spinach 2.1 RNA can bind and activate the fluorophore [http://pubs.acs.org/doi/abs/10.1021/ja410819x DFHBI-1T], both in vivo and in vitro. RNA molecules can thus be fluorescently tagged, by gene fusion with this BioBrick. The flanking tRNA comes from human tRNALys3 and increases stability and folding efficiency of the RNA, leading to higher fluorescent signal. It is possible to detect signal from Spinach2.1 when expressed from moderate strength promoters, e.g. the Anderson promoter library.
This part does not contain or need a Ribosome Binding Site.
Characterization
Spinach2.1 is derived from the Spinach2 sequence described in [http://www.nature.com/nmeth/journal/v10/n12/full/nmeth.2701.html Strack et al. (2013)]. Spinach2.1 has had 2 nucleotides swapped to remove a SpeI site, present in Spinach2.
A folding-prediction is shown below, demonstrating that Spinach2.1 is expected adopt the same fold as Spinach2.
To confirm that Spinach2.1 functions as well as Spinach2, fluorescence measurements were conducted on Spinach2 and Spinach2.1 RNA in vitro. Fluorescence was measured on samples with different concentrations of DFHBI-1T and excess RNA, to compare the brightness of the Spinach-DFHBI-1T complexes. The resulting fluorescence standard curves are shown below:
The slopes of the curves represent the brightness of the RNA-DFHBI-1T complex. The slopes are similar, although Spinach2.1 might be moderately less bright. More experiments need to be conducted to establish whether the difference is significant.
To assess folding efficiency of Spinach2.1 fluorescence was measured on samples with excess DFHBI-1T. Fluorescence normalized to RNA concentration is shown below:
As seen from the chart, there was no significant difference between fluorescence from the two RNA's. This means that equal fractions of RNA was bound to DFHBI-1T, indicating equal folding efficiency.
See [http://2014.igem.org/Team:DTU-Denmark/Achievements/Experimental_Results#lab-comparison-div our wiki] for more details.
2018 Team Hong_Kong-CUHK's Improvement
Spinach2.1 was originally constructed to prevent the illegal site in the superfolding Spinach2. However, its activity might be moderately less bright. On the other hand, Spinach2 has the melting temperature of ~38 degrees Celsius according to previous literature, which might not favor the measurement of heat-induced promoter activity. Comparing the 3 RNA reporters we constructed and the pre-existed Spinach2.1, iSpinach-D5 is moderately brighter.
Collaborating with NUS-A team, we also observed that iSpinach-D5 is moderately more resistant to 37->45 degrees Celsius change than Spinach2.1. However, it was also more vulnerable to 37->30 degrees Celsius change.
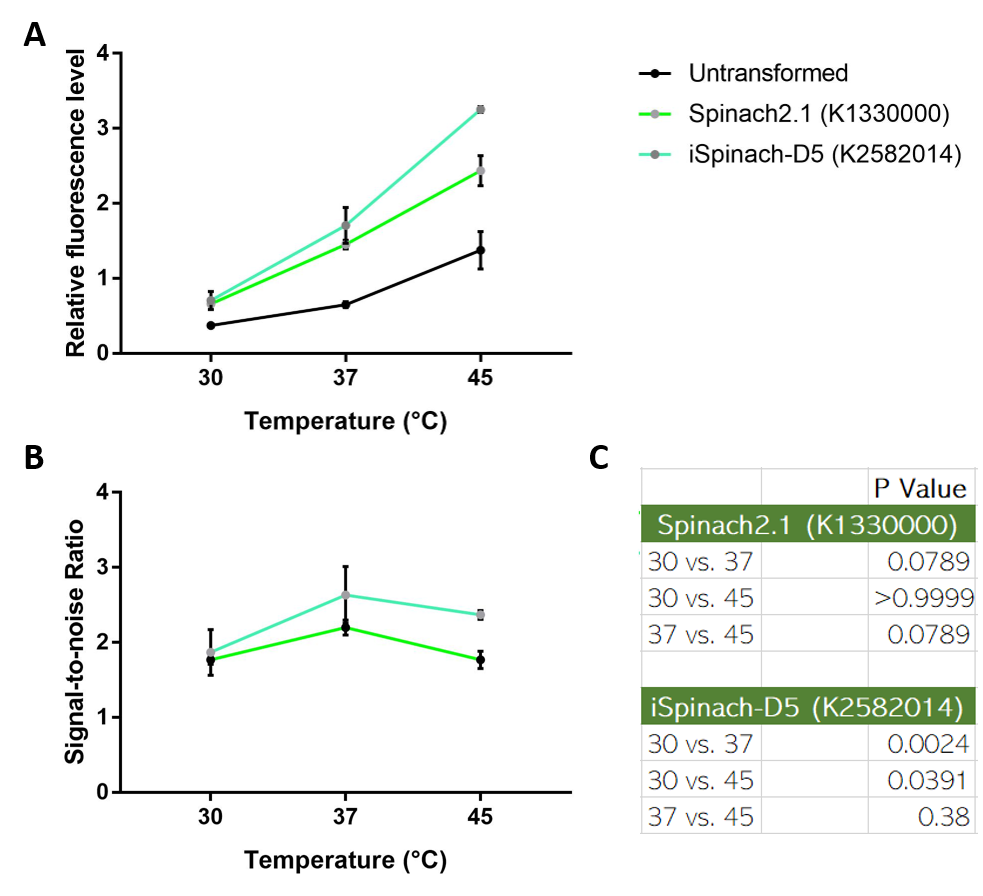
2022 Team IISER-Tirupati_India's Contribution
Potential Spinach 2.1 mutants using Molecular Dynamic Simulations and MFE calculations with higher fluorescence intensity
Towards the modification of parts from the Registry of Standard Biological Parts (RSBP), we aimed to design investigations that would enhance the fluorescence intensity of Spinach 2.1(BBa_K130000), a light-up aptamer. In order to achieve this, we altered the sequence by introducing mutations (single, double), inversions, and deletions at different regions of the Spinach2.1 aptamer sequence to increase its relative fluorescence intensity than the wild-type.
All possible single-point mutations (SPMs) were made in the tetraloop using combinatorics and inversions. The free energy of the thermodynamic ensembles, frequency of the minimum free energy (MFE) structure, and the ensemble diversity were predicted using RNAfold. Based on these predictions, we chose a few sequences that displayed the best parameters for the above predictions. The mutated sequences and their corresponding RNAfold structures are given Figure 1.
Mutations and Inversions:
The free energy of the thermodynamic ensemble are predicted to be -34.44 kcal/mol, -34.44 kcal/mol, -34.80 kcal/mol and -35.50 kcal/mol for the sequences (modified Spinach2.1) of Spinach2.2(BBa_K4438200), Spinach2.3(BBa_K4438201), Spinach2.4(BBa_K4438202) and Spinach2.5(BBa_K4438203) respectively.
Sequence and structures of the RNA Aptamers. The DNA sequence and the RNAfold structures of Spinach2 and Spinach2.1 are adopted from the parts registry made by DTU, Denmark. The Spinach2 sequences highlighted in bold are the aptamer sequence, and those flanking are the tRNA scaffold sequence, tRNALys3. The yellow colored box highlights the tetraloop sequence (colored red) and the bases adjacent (colored blue) to the tetraloop. The bases colored green are mutated from A/T and T/A to give a modified Spinach2 version, Spinach2.1. The other Spinach aptamer versions are derived from the Spinach2 sequence by base mutations in the tetraloop and the region adjacent to it (highlighted in the yellow colored boxes) and keeping the rest of the sequence unchanged. These mutants correspond to Spinach2.2, Spinach2.3, Spinach2.4 and Spinach2.5. The mutations in the DNA sequence are displayed on the RNAfold structures of the RNA aptamers (Spinach). The tetraloop region is indicated by a box colored blue for Spinach2 and the other mutations are circled in blue for other modified Spinach aptamers.
Using these sequences we ran GROMACS simulation to find the energy minimization results. These results were plotted using the steepest descent algorithm and it was observed that the native structure converged in almost 200 steps (Figure 4a) while for Spinach2.6 it converged in nearly 180 steps (Figure 4d). An NVT equilibration was performed and trajectory files were generated to visualise the simulation. It was found that the system reached equilibrium at less than 20 ps (Figure 4b, e). This equilibrated system was subjected to the Steer MD simulations to dislocate the ligand from the G-Quadruplex binding site with spring force 10000 KJ/mol/nm2, see the Figure 4c, d. The force applied was able to pull the ligand apart from the binding site of the RNA aptamer as seen in the animation below. Overall, the energy minimization plot suggests that the Spinach2.6 complex structure is relatively stable when compared with the native structure. Moreover, the Steer MD plots for both these complexes are significantly different suggesting the binding affinities of the ligand to RNA aptamer structure are different.
Results of MD simulations of native and modified RNA aptamers:a, b) Energy minimization and equilibration plots of native (4TS2) complexes respectively. c) Steer MD simulation plot for the native structure. d, e) Energy minimization and equilibration plots of modified Spinach RNA aptamer, Spinach2.6 complex structure respectively and f) Steer MD simulation plot for the Spinach2.6 complex structure
Sequence and Features
- 10COMPATIBLE WITH RFC[10]
- 12COMPATIBLE WITH RFC[12]
- 21COMPATIBLE WITH RFC[21]
- 23COMPATIBLE WITH RFC[23]
- 25COMPATIBLE WITH RFC[25]
- 1000COMPATIBLE WITH RFC[1000]