Difference between revisions of "Part:BBa K3758233"
(→Results) |
|||
(7 intermediate revisions by one other user not shown) | |||
Line 1: | Line 1: | ||
− | |||
__NOTOC__ | __NOTOC__ | ||
− | {|width=' | + | <partinfo>BBa_K3758233 short</partinfo> |
+ | |||
+ | {|width='100%' | ||
|- | |- | ||
|width=35% valign='top'| | |width=35% valign='top'| | ||
− | [[Image: | + | __TOC__ |
+ | | width=80% | | ||
+ | | style="text-align:center;" | '''Secondary Structure''' | ||
+ | [[Image:T--Marburg--rbcL 3'UTR Cr long secondary-structure.png|300px|thumb|prediction of the minimum free energy structure and base pair probability from rbcL 3'UTR of <i>Chlamydomonas</i> using [http://rna.tbi.univie.ac.at/ ViennaRNA]]] | ||
+ | |} | ||
+ | |||
+ | |||
+ | <html> | ||
+ | <style> | ||
+ | .mw-body a.external, .link-https { padding-right: 0 } | ||
+ | #bodyContent a[href^="https://"], .link-https { padding-right: 0 } | ||
+ | </style> | ||
+ | </html> | ||
+ | |||
+ | |||
+ | ==General information== | ||
+ | <p> | ||
+ | This part was created using the Phytobrick Entry Vector with GFP dropout [https://parts.igem.org/Part:BBa_K2560002 BBa_K2560002] and was designed to be compatible with the Phytobrick assembly standard. All parts created this year were acquired via PCR from purified DNA samples using a CTAB based method <sup>[https://doi.org/10.1186/s42269-019-0066-1 <nowiki>[1]</nowiki>]</sup>, by primer annealing, primer annealing, and extension reactions or synthesized via IDT/Twist.</p> | ||
+ | |||
+ | |||
+ | <p> | ||
+ | All parts this year were produced to be used in the chloroplast of different plant species. For the characterization of these parts they were tested in chloroplast cell-free systems (ccfs) from either the same species or they were tested in ccfs from other plant species. Plastid parts offer the benefit of highly conserved regulatory sequences that can be used across species. Although characterizing chloroplast parts is a huge effort, in literature, it has been shown that plastid parts can be used across species to drive gene expression <sup>[https://doi.org/10.7554/eLife.13664 <nowiki>[2]</nowiki>]</sup>. We believe that based on this knowledge we can create valuable parts that can be screened for activity in our system with the final goal of building a variety of different parts. This collection shall help combat unwanted recombination events in vivo that sometimes impede the successful functionality of the genetic design. | ||
+ | </p> | ||
+ | |||
+ | ===3’UTR=== | ||
+ | |||
+ | <p> | ||
+ | 3’ untranslated regions do not function as efficient transcription terminators. They rather form stem-loop RNA secondary structures that are thought to protect the mature RNA from 3’-5’ exoribonucleases. While 3’UTRs play a strong role in the accumulation of RNA transcripts <sup>[https://doi.org/10.1007/s11103-010-9689-1 <nowiki>[11]</nowiki>]</sup>, their effect on protein accumulation is in most cases rather limited <sup>[https://doi.org/10.1046/j.1365-313x.2002.01340.x <nowiki>[12]</nowiki>]</sup>. Nonetheless, under specific conditions and depending on the protein that is planned to be expressed, the choice of the 3’UTR may play a bigger role in protein expression. | ||
+ | Similarly to the 5’UTR, some 3’UTRs are trimmed by specific pentatricopeptide repeat (PPR) proteins that are imported from the nucleus of the plant <sup>[https://doi.org/10.1038/emboj.2009.121 <nowiki>[13]</nowiki>]</sup>. | ||
+ | </p> | ||
+ | |||
+ | ==Characterization & Measurement== | ||
+ | |||
+ | <h3>Batch effect</h3> | ||
+ | |||
+ | <p> | ||
+ | A major difficulty when working with cell free technology is the reproducibility of reliable data generation. While one batch of cell free extract generation might be perfectly suited for efficient protein production, other batches might not perform that well. Because our chloroplast extracts were prepared from leaves, each preparation could have contained distinct compositions of differentiated leaf cells (for example: | ||
+ | mesophyll, palisade parenchyma and bundle sheath) from plants that experienced microclimatic variations. This in turns causes the individual difference in expression strength, making it impossible to quantitatively compare data across measurements. | ||
+ | |||
+ | |||
+ | </p> | ||
+ | |||
+ | <h3>Dual Luciferase normalization</h3> | ||
+ | <p> | ||
+ | To tackle the aforementioned issue, we designed our measurement construct to harbor two individual luciferase cassettes. A standardized cassette that includes the Firefly luciferase (Fluc) is included in every measurement construct in order to have a reference point to which we can normalize our gained data to<sup>[https://doi.org/10.1038/nmeth.3659 <nowiki>[14]</nowiki>] </sup>. | ||
+ | The second cassette consists of a Nano Luciferase (NLuc) harboring a placeholder part in either the promoter, 5’UTR or 3’UTR position. This enables quick exchange of a series of lvl0 parts allowing for high throughput characterization of genetic parts. | ||
+ | |||
+ | </p> | ||
+ | |||
+ | |||
+ | |||
+ | <h3>Placeholder</h3> | ||
+ | |||
+ | <p> | ||
+ | For the characterization of the parts produced this year, we made use of Golden Gate placeholder parts introduced by [http://2019.igem.org/Team:Marburg iGEM Marburg 2019]: | ||
+ | [https://parts.igem.org/Part:BBa_K3228060 BBa_K3228060], | ||
+ | [https://parts.igem.org/Part:BBa_K3228061 BBa_K3228061] and | ||
+ | [https://parts.igem.org/Part:BBa_K3228063 BBa_K3228063]. These placeholder parts can be used in assemblies to subsequently replace it with another part of the same type in a secondary Golden Gate assembly. A placeholder can rationalize large-scale assemblies: Instead of building each plasmid from scratch, a placeholder is used to generate an entry vector. | ||
+ | |||
+ | </p> | ||
+ | |||
+ | |||
+ | |||
+ | |||
+ | ==Results== | ||
+ | |||
+ | <h4>iGEM Marburg 2021 - Improvement of an Existing Part</h4> | ||
+ | <h5>Motivation</h5> | ||
+ | <p> | ||
+ | Cell-free technology offers the possibility for high-throughput characterization of genetic parts in a shorter time frame. This timecut is notable when working with plants in general, but is even more of a hurdle when working on the chloroplast. Normally, it takes several months after a genetic device is introduced until it can be qualitatively/quantitatively characterized. The integration of a transgene in the chloroplast of an organism of choice includes very costly lab equipment and consumables. On top of this, the methods do not offer support to test multiple genetic devices in a time-efficient manner and for many plant species, no chloroplast transformation protocols are available to date. | ||
+ | </p> | ||
+ | <p> | ||
+ | Currently, the repertoire of available genetic parts in the field of chloroplast plant synthetic biology is hugely limited and the characterisation of these tools is very cost-intensive and time consuming. Although a handful of inducible parts and regulatory sequences exist [https://doi.org/10.1111/j.1365-313X.2005.02495.x<nowiki>[17]</nowiki>] [https://doi.org/10.1073/pnas.0914423107<nowiki>[18]</nowiki>] [https://doi.org/10.1073/pnas.0704205104<nowiki>[19]</nowiki>] [https://doi.org/10.1038/s41477-019-0412-1<nowiki>[20]</nowiki>], the small collection poses a huge hurdle for more complex engineering projects. In literature, a lot of ambitious and aspirational projects have been proposed like the implementation of nitrogen fixation pathways into plants or increasing nutritional value in plants [https://doi.org/10.1002/cbic.201900784<nowiki>[21]</nowiki>] [https://dx.doi.org/10.1111%2F1751-7915.12764<nowiki>[22]</nowiki>]. But when considering the limited availability of parts, projects like these seem impossible at the current time. | ||
+ | </p> | ||
+ | <h5>Our Part of Choice</h5> | ||
+ | <p> | ||
+ | The iGEM registry is one of the cornerstones of synthetic biology as it encompasses a collection of over 20.000 parts. As it is normal for iGEM projects to not be completely finished until the end of the iGEM season, we aimed to connect to the efforts of [http://2016.igem.org/Team:Cambridge-JIC iGEM Cambridge 2016]. They introduced a chloroplast part collection for the model algae <i>Chlamydomonas reinhardtii</i>. The toolbox included regulatory sequences, reporter genes, a CRISPR/Cas9 system and a set of homology flanks for genomic integration. As we think it is of high priority to connect to older iGEM projects and reiterate designs during future projects, we decided to have a deeper look into their parts. | ||
+ | During a closer inspection we noticed that the 3’UTR of the rbcL gene (large subunit of the RuBisCo gene) [https://parts.igem.org/Part:BBa_K2148016 BBa_K2148016] BioBrick of their collection was falsely linked to one of their other parts [https://parts.igem.org/Part:BBa_K2148007 BBa_K2148007]. Shortly after, we informed Vinoo Selvarajah head of the iGEM registry about this and consequently the part’s annotation has been corrected. | ||
+ | </p> | ||
+ | <h5>Analysis of the existing part</h5> | ||
+ | <p> | ||
+ | When having a closer look at the part sequence, we noticed remnants of earlier cloning steps. Apart from the 3’UTR region of the rbcL, a large portion of the coding sequence and fragments of the original vector have been included in the part[https://dx.doi.org/10.1099%2Fmic.0.000910<nowiki>[23]</nowiki>], which they took from the cloning vector pASapI [https://doi.org/10.1007/978-1-62703-995-6_27<nowiki>[24]</nowiki>]. | ||
+ | According to our definition of synthetic biology: The foundation of synthetic biology lies in applying engineering principles to biology and one of the key aspects for that is standardization, which is especially important for the creation of standardized biological parts. | ||
+ | As we are dedicated to standardization, we wanted to check if the part would still be functional if we only include the rbcL 3’UTR region and if we detect any significant change in expression when we use different lengths of the 3’UTR. | ||
+ | <br> | ||
+ | With this in mind we took our 3’UTR measurement construct with which we can easily characterize 3’UTR sequences in a high-throughput manner. We therefore exchanged the inserted 3’UTR dropout cassette - a MoClo compatible GFP placeholder introduced by iGEM Marburg 2019 - with the 3 versions of the 3’UTR of <i>Chlamydomonas reinhardtii</i> and tested them in Tobacco chloroplast cell-free extracts in order to test their viability. | ||
+ | <br> | ||
+ | But before conducting the actual experiment we wanted to compare the secondary structure of the different 3’UTRs to see if any significant stem structures could be detected (Figure 1,2,3) | ||
+ | </p> | ||
+ | |||
+ | <html> | ||
+ | <center> | ||
+ | <figure> | ||
+ | <img src='https://2021.igem.org/wiki/images/e/e3/T--Marburg--rbcL_3%27UTR_Cr_BBa_K2148016.png' width='600px' alt='prediction_of_the_secondary_structure_of_BBa_K2148016' /> | ||
+ | <figcaption><b>Figure 1: Prediction of the secondary structure of the BioBrick <a href="https://parts.igem.org/Part:BBa_K2148016">BBa_K2148016</a> of iGEM Cambridge using <a href="http://rna.tbi.univie.ac.at/cgi-bin/RNAWebSuite/RNAfold.cgi">ViennaRNA RNAfold</a> | ||
+ | </b><br> | ||
+ | The part forms a complex structure due to its length. At the 3’ end, a long stem loop structure can be seen(marked by an arrow), which the algorithm could not confidently predict. | ||
+ | </figcaption> | ||
+ | </figure></center></html> | ||
+ | |||
+ | <html> | ||
+ | <center> | ||
+ | <figure> | ||
+ | <img src='https://parts.igem.org/wiki/images/1/16/T--Marburg--rbcL_3%27UTR_Cr_long_secondary-structure.png' width='600px' alt='prediction_of_the_secondary_structure_of_BBa_K3758233' /> | ||
+ | <figcaption><b>Figure 2: Prediction of the secondary structure of our long version of the 3’UTR rbcL Cr using using <a href="http://rna.tbi.univie.ac.at/cgi-bin/RNAWebSuite/RNAfold.cgi">ViennaRNA RNAfold</a> | ||
+ | </b><br> | ||
+ | The part forms a complex structure with two strong distinct stem loop structures near the beginning of the 5’ end of the 3’UTR (1). Another strong distinct structure can be seen(2), which is a tRNA we included in the part for potential higher expression | ||
+ | </figcaption> | ||
+ | </figure></center></html> | ||
+ | |||
+ | <html> | ||
+ | <center> | ||
+ | <figure> | ||
+ | <img src='https://parts.igem.org/wiki/images/f/f1/T--Marburg--rbcL_3%27UTR_Cr_short_secondary-structure.png' width='600px' alt='prediction_of_the_secondary_structure_of_BBa_K3758234' /> | ||
+ | <figcaption><b>Figure 3: Prediction of the secondary structure of our short version of the 3’UTR rbcL Cr using <a href="http://rna.tbi.univie.ac.at/cgi-bin/RNAWebSuite/RNAfold.cgi">ViennaRNA RNAfold</a> | ||
+ | </b><br> | ||
+ | A strong stem loop structure can be detected. This is the short version we designed, because we noticed a huge drop in our Transcriptomic data set directly upstream of this stem loop | ||
+ | </figcaption> | ||
+ | </figure></center></html> | ||
+ | |||
+ | <h5>Comparison of the 3'UTR versions</h5> | ||
+ | <p> | ||
+ | 3’ untranslated regions in chloroplasts do not necessarily function as efficient transcription terminators. They rather form distinct secondary structures that stabilizes the RNA and protects it from 3’ endonucleases. During our research on the 3’UTR of rbcL from <i>Chlamydomonas reinhardtii</i> we found out that in fact 60% of transcription does not terminate at the 3’UTR region, but instead continues downstream [https://doi.org/10.1017/s1355838201001479<nowiki>[25]</nowiki>]. On top of this we also found out that there are two distinct stem loops found directly downstream the stopcodon of the rbcL gene that are thought to protect the mature RNA from degradation. These stem loops were clearly visible in the long version of the 3’UTR rbcL. In the short version of the rbcL 3’UTR only the first loop structure is visible. This is due to us designing this part in accordance with our Transcriptomic data set that showed a huge drop in reads directly upstream of the first stem loop structure. | ||
+ | <br><br> | ||
+ | |||
+ | After comparing the different secondary structure predictions we noticed that the model had problems accurately predicting the fold of the BioBrick of Cambridge, while our versions were easier to predict for the algorithm. It was also notable that the longer version showed good stem loop structures, because of the tRNA that was included in the part. The smaller version included a potentially strong stem loop structure that is enveloped by two poly-U regions. | ||
+ | With this in mind we went ahead and conducted the actual measurement. | ||
+ | |||
+ | </p> | ||
+ | |||
+ | <html> | ||
+ | <center> | ||
+ | <figure> | ||
+ | <img src='https://2021.igem.org/wiki/images/d/db/T--Marburg--improve_a_part.png' width='600px' alt='Comparison_of_the_three_3'UTR_versions' /> | ||
+ | <figcaption><b>Figure 4: Comparisons of the different rbcL 3’UTR versions. | ||
+ | |||
+ | </b><br> | ||
+ | Luminescence values are given as arbitrary units and the data is presented on a logarithmic scale. The reaction was set up with a total volume of 10µl. Negative controls using only the plasmid DNA or the crude chloroplast extracts have been included respectively in order to verify the expression. This measurement was performed with the BioBricks <a class="igem" href="https://parts.igem.org/Part:BBa_K2148016">BBa_K2148016</a>, <a class="igem" href="https://parts.igem.org/Part:BBa_K3758233">BBa_K3758233</a> and <a class="igem" href="https://parts.igem.org/Part:BBa_K3758234">BBa_K3758234</a> that were introduced into the 3’UTR measurement lvl2 vector | ||
+ | </figcaption> | ||
+ | </figure></center></html> | ||
+ | |||
+ | <h5>Conclusion</h5> | ||
+ | <p> | ||
+ | On the basis of the data we could not detect any significant difference in expression between the three 3’UTR sequences. Therefore we argue that the short version of the 3'UTR of rbcL of <i>Chlamydomonas</i> is our improved version of the original BioBrick, as the part retains full functionality, while simultaneously getting rid of unnecessary DNA sequences. In accordance with our dedication to standardization, we suggest not to include remnants from older cloning projects in parts as they would accumulate over time and could negatively influence genetic designs in the future. Also, in order to better track the history of parts it might be better to only include sequences that are directly graspable from the parts name. | ||
+ | We therefore present [https://parts.igem.org/Part:BBa_K3758234 BBa_K3758234] as our improved version of the rbcL 3’UTR of <i>Chlamydomonas reinhardtii</i>. | ||
+ | </p> | ||
+ | |||
+ | ==Marburg collection 3.0== | ||
+ | <p> | ||
+ | We proudly present the third expansion of the Marburg collection <sup>[https://doi.org/10.1021/acssynbio.1c00126 <nowiki>[15]</nowiki>]</sup>. The Marburg collection is a Golden Gate based toolbox containing various parts that are compatible with the PhytoBrick system and MoClo. Compared to other bacterial toolboxes, the Marburg Collection shines with superior flexibility. The collection overcame the rigid paradigm of plasmid construction - thinking in fixed backbone and insert categories - by achieving complete de novo assembly of plasmids. | ||
+ | 36 connectors facilitate flexible cloning of multigene constructs and even allow for the inversion of individual transcription units.<br><br> | ||
+ | The original [http://2018.igem.org/Team:Marburg/Part_Collection Marburg Collection] contains 123 parts in total, including: | ||
+ | inducible promoters, reporters, fluorescence and epitope tags, oris, resistance cassettes and genome engineering tools. The toolbox was constructed as a foundation for future iGEM teams to empower accelerated progression in their ambitious projects. | ||
+ | <br><br> | ||
+ | Our collection includes genetic parts suitable for use in the chloroplast. Among parts from the chloroplast of <i>Nicotiana tabacum</i>, we built parts from the chloroplast of <i>Spinacia oleracea</i>, <i>Oryza sativa</i>,<i>Triticum aestivum</i> and <i>Quercus robur</i>. With our contribution, we aim to accelerate research in the field of plastid engineering. | ||
+ | </p> | ||
+ | |||
+ | |||
+ | <span class='h3bb'>Sequence and Features</span> | ||
+ | <partinfo>BBa_K3758233 SequenceAndFeatures</partinfo> | ||
+ | |||
+ | |||
+ | <!--- | ||
+ | ===Functional Parameters=== | ||
<partinfo>BBa_K3758233 parameters</partinfo> | <partinfo>BBa_K3758233 parameters</partinfo> | ||
− | + | <!-- --> | |
− | < | + | |
− | + | ||
− | |||
− | + | ==References== | |
+ | |||
+ | [1] Aboul-Maaty, N. A.-F., & Oraby, H. A.-S. (2019). Extraction of high-quality genomic DNA from different plant orders applying a modified CTAB-based method. Bulletin of the National Research Centre, 43(1). https://doi.org/10.1186/s42269-019-0066-1 | ||
+ | |||
+ | [2] Fuentes, P., Zhou, F., Erban, A., Karcher, D., Kopka, J., & Bock, R. (2016). A new synthetic biology approach allows transfer of an entire metabolic pathway from a medicinal plant to a biomass crop. ELife, 5. https://doi.org/10.7554/elife.13664 | ||
+ | |||
+ | [3] Filée, J., & Forterre, P. (2005). Viral proteins functioning in organelles: a cryptic origin? Trends in Microbiology, 13(11), 510–513. https://doi.org/10.1016/j.tim.2005.08.012 | ||
+ | |||
+ | [4] Liere, K., & Börner, T. (2007). Transcription and transcriptional regulation in plastids. In Cell and Molecular Biology of Plastids (pp. 121–174). Springer Berlin Heidelberg. https://doi.org/10.1007/4735_2007_0232 | ||
+ | |||
+ | [5] Xie, G., & Allison, L. (2002). Sequences upstream of the YRTA core region are essential for transcription of the tobacco atpB NEP promoter in chloroplasts in vivo. Current Genetics, 41(3), 176–182. https://doi.org/10.1007/s00294-002-0293-z | ||
+ | |||
+ | [6] Kuroda, H., & Maliga, P. (2002). Overexpression of the clpP 5′-Untranslated Region in a Chimeric Context Causes a Mutant Phenotype, Suggesting Competition for a clpP-Specific RNA Maturation Factor in Tobacco Chloroplasts. Plant Physiology, 129(4), 1600–1606. https://doi.org/10.1104/pp.004986 | ||
+ | |||
+ | [7] Klinkert, B. (2006). Translation of chloroplast psbD mRNA in Chlamydomonas is controlled by a secondary RNA structure blocking the AUG start codon. Nucleic Acids Research, 34(1), 386–394. https://doi.org/10.1093/nar/gkj433 | ||
+ | |||
+ | [8] Zhelyazkova, P., Hammani, K., Rojas, M., Voelker, R., Vargas-Suárez, M., Börner, T., & Barkan, A. (2011). Protein-mediated protection as the predominant mechanism for defining processed mRNA termini in land plant chloroplasts. Nucleic Acids Research, 40(7), 3092–3105. https://doi.org/10.1093/nar/gkr1137 | ||
+ | |||
+ | [9] Zoschke, R., Kroeger, T., Belcher, S., Schöttler, M. A., Barkan, A., & Schmitz-Linneweber, C. (2012). The pentatricopeptide repeat-SMR protein ATP4 promotes translation of the chloroplastatpB/EmRNA. The Plant Journal, 72(4), 547–558. https://doi.org/10.1111/j.1365-313x.2012.05081.x | ||
+ | |||
+ | [10] Zhang, L., Zhou, W., Che, L., Rochaix, J.-D., Lu, C., Li, W., & Peng, L. (2019). PPR Protein BFA2 Is Essential for the Accumulation of the atpH/F Transcript in Chloroplasts. Frontiers in Plant Science, 10. https://doi.org/10.3389/fpls.2019.00446 | ||
+ | |||
+ | [11] Tangphatsornruang, S., Birch-Machin, I., Newell, C. A., & Gray, J. C. (2010). The effect of different 3′ untranslated regions on the accumulation and stability of transcripts of a gfp transgene in chloroplasts of transplastomic tobacco. Plant Molecular Biology, 76(3–5), 385–396. https://doi.org/10.1007/s11103-010-9689-1 | ||
+ | |||
+ | [12] Eberhard, S., Drapier, D., & Wollman, F.-A. (2002). Searching limiting steps in the expression of chloroplast-encoded proteins: relations between gene copy number, transcription, transcript abundance and translation rate in the chloroplast of Chlamydomonas reinhardtii. The Plant Journal, 31(2), 149–160. https://doi.org/10.1046/j.1365-313x.2002.01340.x | ||
+ | |||
+ | [13] Pfalz, J., Bayraktar, O. A., Prikryl, J., & Barkan, A. (2009). Site-specific binding of a PPR protein defines and stabilizes 5′ and 3′ mRNA termini in chloroplasts. The EMBO Journal, 28(14), 2042–2052. https://doi.org/10.1038/emboj.2009.121 | ||
+ | |||
+ | [14] Schaumberg, K. A., Antunes, M. S., Kassaw, T. K., Xu, W., Zalewski, C. S., Medford, J. I., & Prasad, A. (2015). Quantitative characterization of genetic parts and circuits for plant synthetic biology. Nature Methods, 13(1), 94–100. https://doi.org/10.1038/nmeth.3659 | ||
− | [ | + | [15] Suzuki, J. Y., Sriraman, P., Svab, Z., & Maliga, P. (2003). Unique Architecture of the Plastid Ribosomal RNA Operon Promoter Recognized by the Multisubunit RNA Polymerase in Tobacco and Other Higher Plants. The Plant Cell, 15(1), 195–205. https://doi.org/10.1105/tpc.007914 |
− | + | [16] Occhialini, A., Piatek, A. A., Pfotenhauer, A. C., Frazier, T. P., Stewart, C. N., Jr., & Lenaghan, S. C. (2019). MoChlo: A Versatile, Modular Cloning Toolbox for Chloroplast Biotechnology. Plant Physiology, 179(3), 943–957. https://doi.org/10.1104/pp.18.01220 | |
− | + | ||
− | + |
Latest revision as of 01:26, 20 October 2021
rbcL 3-UTR long (Chlamydomonas reinhardtii)
Secondary Structure |
General information
This part was created using the Phytobrick Entry Vector with GFP dropout BBa_K2560002 and was designed to be compatible with the Phytobrick assembly standard. All parts created this year were acquired via PCR from purified DNA samples using a CTAB based method [1], by primer annealing, primer annealing, and extension reactions or synthesized via IDT/Twist.
All parts this year were produced to be used in the chloroplast of different plant species. For the characterization of these parts they were tested in chloroplast cell-free systems (ccfs) from either the same species or they were tested in ccfs from other plant species. Plastid parts offer the benefit of highly conserved regulatory sequences that can be used across species. Although characterizing chloroplast parts is a huge effort, in literature, it has been shown that plastid parts can be used across species to drive gene expression [2]. We believe that based on this knowledge we can create valuable parts that can be screened for activity in our system with the final goal of building a variety of different parts. This collection shall help combat unwanted recombination events in vivo that sometimes impede the successful functionality of the genetic design.
3’UTR
3’ untranslated regions do not function as efficient transcription terminators. They rather form stem-loop RNA secondary structures that are thought to protect the mature RNA from 3’-5’ exoribonucleases. While 3’UTRs play a strong role in the accumulation of RNA transcripts [11], their effect on protein accumulation is in most cases rather limited [12]. Nonetheless, under specific conditions and depending on the protein that is planned to be expressed, the choice of the 3’UTR may play a bigger role in protein expression. Similarly to the 5’UTR, some 3’UTRs are trimmed by specific pentatricopeptide repeat (PPR) proteins that are imported from the nucleus of the plant [13].
Characterization & Measurement
Batch effect
A major difficulty when working with cell free technology is the reproducibility of reliable data generation. While one batch of cell free extract generation might be perfectly suited for efficient protein production, other batches might not perform that well. Because our chloroplast extracts were prepared from leaves, each preparation could have contained distinct compositions of differentiated leaf cells (for example: mesophyll, palisade parenchyma and bundle sheath) from plants that experienced microclimatic variations. This in turns causes the individual difference in expression strength, making it impossible to quantitatively compare data across measurements.
Dual Luciferase normalization
To tackle the aforementioned issue, we designed our measurement construct to harbor two individual luciferase cassettes. A standardized cassette that includes the Firefly luciferase (Fluc) is included in every measurement construct in order to have a reference point to which we can normalize our gained data to[14] . The second cassette consists of a Nano Luciferase (NLuc) harboring a placeholder part in either the promoter, 5’UTR or 3’UTR position. This enables quick exchange of a series of lvl0 parts allowing for high throughput characterization of genetic parts.
Placeholder
For the characterization of the parts produced this year, we made use of Golden Gate placeholder parts introduced by [http://2019.igem.org/Team:Marburg iGEM Marburg 2019]: BBa_K3228060, BBa_K3228061 and BBa_K3228063. These placeholder parts can be used in assemblies to subsequently replace it with another part of the same type in a secondary Golden Gate assembly. A placeholder can rationalize large-scale assemblies: Instead of building each plasmid from scratch, a placeholder is used to generate an entry vector.
Results
iGEM Marburg 2021 - Improvement of an Existing Part
Motivation
Cell-free technology offers the possibility for high-throughput characterization of genetic parts in a shorter time frame. This timecut is notable when working with plants in general, but is even more of a hurdle when working on the chloroplast. Normally, it takes several months after a genetic device is introduced until it can be qualitatively/quantitatively characterized. The integration of a transgene in the chloroplast of an organism of choice includes very costly lab equipment and consumables. On top of this, the methods do not offer support to test multiple genetic devices in a time-efficient manner and for many plant species, no chloroplast transformation protocols are available to date.
Currently, the repertoire of available genetic parts in the field of chloroplast plant synthetic biology is hugely limited and the characterisation of these tools is very cost-intensive and time consuming. Although a handful of inducible parts and regulatory sequences exist [17] [18] [19] [20], the small collection poses a huge hurdle for more complex engineering projects. In literature, a lot of ambitious and aspirational projects have been proposed like the implementation of nitrogen fixation pathways into plants or increasing nutritional value in plants [21] [22]. But when considering the limited availability of parts, projects like these seem impossible at the current time.
Our Part of Choice
The iGEM registry is one of the cornerstones of synthetic biology as it encompasses a collection of over 20.000 parts. As it is normal for iGEM projects to not be completely finished until the end of the iGEM season, we aimed to connect to the efforts of [http://2016.igem.org/Team:Cambridge-JIC iGEM Cambridge 2016]. They introduced a chloroplast part collection for the model algae Chlamydomonas reinhardtii. The toolbox included regulatory sequences, reporter genes, a CRISPR/Cas9 system and a set of homology flanks for genomic integration. As we think it is of high priority to connect to older iGEM projects and reiterate designs during future projects, we decided to have a deeper look into their parts. During a closer inspection we noticed that the 3’UTR of the rbcL gene (large subunit of the RuBisCo gene) BBa_K2148016 BioBrick of their collection was falsely linked to one of their other parts BBa_K2148007. Shortly after, we informed Vinoo Selvarajah head of the iGEM registry about this and consequently the part’s annotation has been corrected.
Analysis of the existing part
When having a closer look at the part sequence, we noticed remnants of earlier cloning steps. Apart from the 3’UTR region of the rbcL, a large portion of the coding sequence and fragments of the original vector have been included in the part[23], which they took from the cloning vector pASapI [24].
According to our definition of synthetic biology: The foundation of synthetic biology lies in applying engineering principles to biology and one of the key aspects for that is standardization, which is especially important for the creation of standardized biological parts.
As we are dedicated to standardization, we wanted to check if the part would still be functional if we only include the rbcL 3’UTR region and if we detect any significant change in expression when we use different lengths of the 3’UTR.
With this in mind we took our 3’UTR measurement construct with which we can easily characterize 3’UTR sequences in a high-throughput manner. We therefore exchanged the inserted 3’UTR dropout cassette - a MoClo compatible GFP placeholder introduced by iGEM Marburg 2019 - with the 3 versions of the 3’UTR of Chlamydomonas reinhardtii and tested them in Tobacco chloroplast cell-free extracts in order to test their viability.
But before conducting the actual experiment we wanted to compare the secondary structure of the different 3’UTRs to see if any significant stem structures could be detected (Figure 1,2,3)
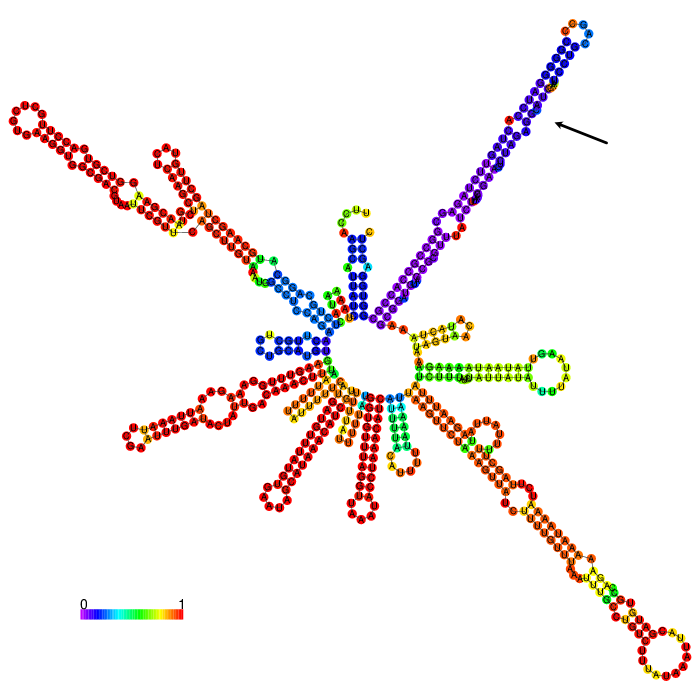
The part forms a complex structure due to its length. At the 3’ end, a long stem loop structure can be seen(marked by an arrow), which the algorithm could not confidently predict.
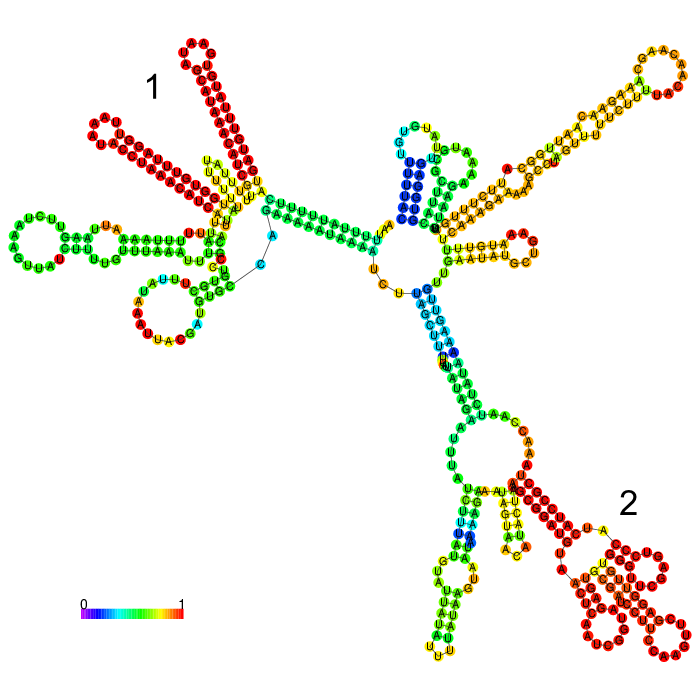
The part forms a complex structure with two strong distinct stem loop structures near the beginning of the 5’ end of the 3’UTR (1). Another strong distinct structure can be seen(2), which is a tRNA we included in the part for potential higher expression

A strong stem loop structure can be detected. This is the short version we designed, because we noticed a huge drop in our Transcriptomic data set directly upstream of this stem loop
Comparison of the 3'UTR versions
3’ untranslated regions in chloroplasts do not necessarily function as efficient transcription terminators. They rather form distinct secondary structures that stabilizes the RNA and protects it from 3’ endonucleases. During our research on the 3’UTR of rbcL from Chlamydomonas reinhardtii we found out that in fact 60% of transcription does not terminate at the 3’UTR region, but instead continues downstream [25]. On top of this we also found out that there are two distinct stem loops found directly downstream the stopcodon of the rbcL gene that are thought to protect the mature RNA from degradation. These stem loops were clearly visible in the long version of the 3’UTR rbcL. In the short version of the rbcL 3’UTR only the first loop structure is visible. This is due to us designing this part in accordance with our Transcriptomic data set that showed a huge drop in reads directly upstream of the first stem loop structure.
After comparing the different secondary structure predictions we noticed that the model had problems accurately predicting the fold of the BioBrick of Cambridge, while our versions were easier to predict for the algorithm. It was also notable that the longer version showed good stem loop structures, because of the tRNA that was included in the part. The smaller version included a potentially strong stem loop structure that is enveloped by two poly-U regions.
With this in mind we went ahead and conducted the actual measurement.

Luminescence values are given as arbitrary units and the data is presented on a logarithmic scale. The reaction was set up with a total volume of 10µl. Negative controls using only the plasmid DNA or the crude chloroplast extracts have been included respectively in order to verify the expression. This measurement was performed with the BioBricks BBa_K2148016, BBa_K3758233 and BBa_K3758234 that were introduced into the 3’UTR measurement lvl2 vector
Conclusion
On the basis of the data we could not detect any significant difference in expression between the three 3’UTR sequences. Therefore we argue that the short version of the 3'UTR of rbcL of Chlamydomonas is our improved version of the original BioBrick, as the part retains full functionality, while simultaneously getting rid of unnecessary DNA sequences. In accordance with our dedication to standardization, we suggest not to include remnants from older cloning projects in parts as they would accumulate over time and could negatively influence genetic designs in the future. Also, in order to better track the history of parts it might be better to only include sequences that are directly graspable from the parts name. We therefore present BBa_K3758234 as our improved version of the rbcL 3’UTR of Chlamydomonas reinhardtii.
Marburg collection 3.0
We proudly present the third expansion of the Marburg collection [15]. The Marburg collection is a Golden Gate based toolbox containing various parts that are compatible with the PhytoBrick system and MoClo. Compared to other bacterial toolboxes, the Marburg Collection shines with superior flexibility. The collection overcame the rigid paradigm of plasmid construction - thinking in fixed backbone and insert categories - by achieving complete de novo assembly of plasmids.
36 connectors facilitate flexible cloning of multigene constructs and even allow for the inversion of individual transcription units.
The original [http://2018.igem.org/Team:Marburg/Part_Collection Marburg Collection] contains 123 parts in total, including:
inducible promoters, reporters, fluorescence and epitope tags, oris, resistance cassettes and genome engineering tools. The toolbox was constructed as a foundation for future iGEM teams to empower accelerated progression in their ambitious projects.
Our collection includes genetic parts suitable for use in the chloroplast. Among parts from the chloroplast of Nicotiana tabacum, we built parts from the chloroplast of Spinacia oleracea, Oryza sativa,Triticum aestivum and Quercus robur. With our contribution, we aim to accelerate research in the field of plastid engineering.
Sequence and Features
- 10COMPATIBLE WITH RFC[10]
- 12COMPATIBLE WITH RFC[12]
- 21COMPATIBLE WITH RFC[21]
- 23COMPATIBLE WITH RFC[23]
- 25COMPATIBLE WITH RFC[25]
- 1000COMPATIBLE WITH RFC[1000]
References
[1] Aboul-Maaty, N. A.-F., & Oraby, H. A.-S. (2019). Extraction of high-quality genomic DNA from different plant orders applying a modified CTAB-based method. Bulletin of the National Research Centre, 43(1). https://doi.org/10.1186/s42269-019-0066-1
[2] Fuentes, P., Zhou, F., Erban, A., Karcher, D., Kopka, J., & Bock, R. (2016). A new synthetic biology approach allows transfer of an entire metabolic pathway from a medicinal plant to a biomass crop. ELife, 5. https://doi.org/10.7554/elife.13664
[3] Filée, J., & Forterre, P. (2005). Viral proteins functioning in organelles: a cryptic origin? Trends in Microbiology, 13(11), 510–513. https://doi.org/10.1016/j.tim.2005.08.012
[4] Liere, K., & Börner, T. (2007). Transcription and transcriptional regulation in plastids. In Cell and Molecular Biology of Plastids (pp. 121–174). Springer Berlin Heidelberg. https://doi.org/10.1007/4735_2007_0232
[5] Xie, G., & Allison, L. (2002). Sequences upstream of the YRTA core region are essential for transcription of the tobacco atpB NEP promoter in chloroplasts in vivo. Current Genetics, 41(3), 176–182. https://doi.org/10.1007/s00294-002-0293-z
[6] Kuroda, H., & Maliga, P. (2002). Overexpression of the clpP 5′-Untranslated Region in a Chimeric Context Causes a Mutant Phenotype, Suggesting Competition for a clpP-Specific RNA Maturation Factor in Tobacco Chloroplasts. Plant Physiology, 129(4), 1600–1606. https://doi.org/10.1104/pp.004986
[7] Klinkert, B. (2006). Translation of chloroplast psbD mRNA in Chlamydomonas is controlled by a secondary RNA structure blocking the AUG start codon. Nucleic Acids Research, 34(1), 386–394. https://doi.org/10.1093/nar/gkj433
[8] Zhelyazkova, P., Hammani, K., Rojas, M., Voelker, R., Vargas-Suárez, M., Börner, T., & Barkan, A. (2011). Protein-mediated protection as the predominant mechanism for defining processed mRNA termini in land plant chloroplasts. Nucleic Acids Research, 40(7), 3092–3105. https://doi.org/10.1093/nar/gkr1137
[9] Zoschke, R., Kroeger, T., Belcher, S., Schöttler, M. A., Barkan, A., & Schmitz-Linneweber, C. (2012). The pentatricopeptide repeat-SMR protein ATP4 promotes translation of the chloroplastatpB/EmRNA. The Plant Journal, 72(4), 547–558. https://doi.org/10.1111/j.1365-313x.2012.05081.x
[10] Zhang, L., Zhou, W., Che, L., Rochaix, J.-D., Lu, C., Li, W., & Peng, L. (2019). PPR Protein BFA2 Is Essential for the Accumulation of the atpH/F Transcript in Chloroplasts. Frontiers in Plant Science, 10. https://doi.org/10.3389/fpls.2019.00446
[11] Tangphatsornruang, S., Birch-Machin, I., Newell, C. A., & Gray, J. C. (2010). The effect of different 3′ untranslated regions on the accumulation and stability of transcripts of a gfp transgene in chloroplasts of transplastomic tobacco. Plant Molecular Biology, 76(3–5), 385–396. https://doi.org/10.1007/s11103-010-9689-1
[12] Eberhard, S., Drapier, D., & Wollman, F.-A. (2002). Searching limiting steps in the expression of chloroplast-encoded proteins: relations between gene copy number, transcription, transcript abundance and translation rate in the chloroplast of Chlamydomonas reinhardtii. The Plant Journal, 31(2), 149–160. https://doi.org/10.1046/j.1365-313x.2002.01340.x
[13] Pfalz, J., Bayraktar, O. A., Prikryl, J., & Barkan, A. (2009). Site-specific binding of a PPR protein defines and stabilizes 5′ and 3′ mRNA termini in chloroplasts. The EMBO Journal, 28(14), 2042–2052. https://doi.org/10.1038/emboj.2009.121
[14] Schaumberg, K. A., Antunes, M. S., Kassaw, T. K., Xu, W., Zalewski, C. S., Medford, J. I., & Prasad, A. (2015). Quantitative characterization of genetic parts and circuits for plant synthetic biology. Nature Methods, 13(1), 94–100. https://doi.org/10.1038/nmeth.3659
[15] Suzuki, J. Y., Sriraman, P., Svab, Z., & Maliga, P. (2003). Unique Architecture of the Plastid Ribosomal RNA Operon Promoter Recognized by the Multisubunit RNA Polymerase in Tobacco and Other Higher Plants. The Plant Cell, 15(1), 195–205. https://doi.org/10.1105/tpc.007914
[16] Occhialini, A., Piatek, A. A., Pfotenhauer, A. C., Frazier, T. P., Stewart, C. N., Jr., & Lenaghan, S. C. (2019). MoChlo: A Versatile, Modular Cloning Toolbox for Chloroplast Biotechnology. Plant Physiology, 179(3), 943–957. https://doi.org/10.1104/pp.18.01220