Difference between revisions of "Part:BBa K3782000"
(2 intermediate revisions by the same user not shown) | |||
Line 38: | Line 38: | ||
<br> | <br> | ||
'''FfIBP''' is naturally produced by the Gram-negative Antarctic bacterium <i>Flavobacterium frigoris</i> PS1. Its TH activity is around 2.5 K at 50 µM<ref>Do H, Lee JH, Lee SG, Kim HJ. Crystallization and preliminary X-ray crystallographic analysis of an ice-binding protein (FfIBP) from Flavobacterium frigoris PS1. Acta Crystallogr Sect F Struct Biol Cryst Commun [Internet]. 2012 Jul [cited 2021 Jul 7];68(Pt 7):806. Available from: /pmc/articles/PMC3388927/</ref>. | '''FfIBP''' is naturally produced by the Gram-negative Antarctic bacterium <i>Flavobacterium frigoris</i> PS1. Its TH activity is around 2.5 K at 50 µM<ref>Do H, Lee JH, Lee SG, Kim HJ. Crystallization and preliminary X-ray crystallographic analysis of an ice-binding protein (FfIBP) from Flavobacterium frigoris PS1. Acta Crystallogr Sect F Struct Biol Cryst Commun [Internet]. 2012 Jul [cited 2021 Jul 7];68(Pt 7):806. Available from: /pmc/articles/PMC3388927/</ref>. | ||
+ | <br> | ||
+ | <br> | ||
+ | Antifreeze proteins bind to ice crystals and inhibit their growth. Ice crystals don’t have the same molecular arrangement on each of its faces and certain AFPs tend to adsorb to the basal plane of the crystals whilst others tend to adsorb to the prism plane. Hyperactive AFPs can bind to every plane<ref>Olijve LLC, Meister K, DeVries AL, Duman JG, Guo S, Bakker HJ, et al. Blocking rapid ice crystal growth through nonbasal plane adsorption of antifreeze proteins. Proc Natl Acad Sci [Internet]. 2016 Apr 5 [cited 2021 Oct 10];113(14):3740–5. Available from: https://www.pnas.org/content/113/14/3740</ref>. | ||
+ | The mechanism by which AFPs suppress the freezing point of a solution still isn’t completely understood. Nevertheless, AFPs are believed to attach irreversibly to the plane of a growing ice crystal. As the protein adsorbs to the growing ice plane, growth at the site is suppressed, producing bulges in between the adsorbed proteins<ref>LM S, AV T. Kinetic pinning and biological antifreezes. Phys Rev Lett [Internet]. 2004 Sep 17 [cited 2021 Oct 10];93(12). Available from: https://pubmed.ncbi.nlm.nih.gov/15447309/</ref>. Due to the Gibbs-Thomson effect, the freezing point will be depressed<ref>Pereyra RG, Szleifer I, Carignano MA. Temperature dependence of ice critical nucleus size. J Chem Phys [Internet]. 2011 Jul 21 [cited 2021 Oct 10];135(3):034508. Available from: https://aip.scitation.org/doi/abs/10.1063/1.3613672</ref>. | ||
</p> | </p> | ||
− | + | ||
=Characterization= | =Characterization= | ||
Line 71: | Line 75: | ||
<br> | <br> | ||
<h3>Expression and Purification</h3> | <h3>Expression and Purification</h3> | ||
− | |||
− | |||
− | |||
[[File:T--UNILausanne--FfIBP SDS.png|400px|thumb|right|'''Figure 2:''' SDS-PAGE electrophoresis before and after FfIBP purification. FfIBP was expressed in E. coli BL21 (DE3) using both pColdI and pET-17b vectors. Left to right: L – PageRuler Plus Prestained Protein Ladder, 10 to 250 kDa (26619), 1 – Bacterial lysate of FfIBP expressed using the pColdI plasmid, 2 – Elution fraction of FfIBP expressed using the pColdI plasmid, 3 – Bacterial lysate of FfIBP expressed using the pET-17b plasmid, 4 – Elution fraction of FfIBP expressed using the pET-17b plasmid.]] | [[File:T--UNILausanne--FfIBP SDS.png|400px|thumb|right|'''Figure 2:''' SDS-PAGE electrophoresis before and after FfIBP purification. FfIBP was expressed in E. coli BL21 (DE3) using both pColdI and pET-17b vectors. Left to right: L – PageRuler Plus Prestained Protein Ladder, 10 to 250 kDa (26619), 1 – Bacterial lysate of FfIBP expressed using the pColdI plasmid, 2 – Elution fraction of FfIBP expressed using the pColdI plasmid, 3 – Bacterial lysate of FfIBP expressed using the pET-17b plasmid, 4 – Elution fraction of FfIBP expressed using the pET-17b plasmid.]] | ||
+ | <br> | ||
<p style="margin-right:2.5em;" align="justify"> | <p style="margin-right:2.5em;" align="justify"> | ||
+ | After we successfully transformed <i>E. coli</i> strain BL21 (DE3) with our pET-17b-FfIBP and pColdI-FfIBP constructs, we inoculated liquid cultures with LB and ampicillin. Before inducing expression in large volumes of liquid cultures, we first did small-scale test purifications to verify if the antifreeze proteins could be correctly purified. Next, we grew 1-liter liquid cultures at 37°C until an OD<sub>600</sub> of 0.6 was reached. We then placed the culture in a cooling incubator at 15°C for 30 minutes. We added IPTG 1mM and induced expression at 15°C for 24 hours. Afterwards, we removed a small volume of culture to run a total cell SDS-PAGE (15%) of E. coli cultures stained with Coomassie blue and to verify that the antifreeze protein was correctly overexpressed. The remaining culture was centrifuged, and the cell pellet was resuspended in Cold Lysis Buffer. We sonicated the cells and centrifuged the cells once more to collect the supernatant, which we loaded into the His-tag affinity column. We eluted the antifreeze proteins which bound to the column and concentrated the solution to a smaller volume. We performed a gel filtration to further purify the protein and finally stored aliquots at -80°C. | ||
+ | <br> | ||
<br> | <br> | ||
We induced expression of FfIBP with IPTG and ran a total cell SDS-PAGE (15%) of the E. coli BL21 (DE3) cultures stained with Coomassie blue. We used two different vectors, pET-17b and pColdI, to compare how the promoters influenced expression efficiency. The overexpression was successful for FfIBP. We observed thicker bands that matched the weight of the protein. The pColdI vector worked better than the pET-17b vector, because the band appeared to be stronger for this first plasmid on the gel (Fig.2). | We induced expression of FfIBP with IPTG and ran a total cell SDS-PAGE (15%) of the E. coli BL21 (DE3) cultures stained with Coomassie blue. We used two different vectors, pET-17b and pColdI, to compare how the promoters influenced expression efficiency. The overexpression was successful for FfIBP. We observed thicker bands that matched the weight of the protein. The pColdI vector worked better than the pET-17b vector, because the band appeared to be stronger for this first plasmid on the gel (Fig.2). | ||
</p> | </p> | ||
<br> | <br> | ||
+ | <h3>ISF Assay</h3> | ||
+ | [[File:T--UNILausanne--AFPs_TH.png|400px|thumb|right|'''Figure 3:''' Thermal hysteresis of RiAFP and FfIBP increase with their concentration. Connected scatter plot graph showing the mean of biological triplicate +/- standard deviation. Thermal hysteresis is measured with two different AFPs, RiAFP and FfIBP, at different concentrations (µM).]] | ||
<br> | <br> | ||
+ | <p style="margin-right:2.5em;" align="justify"> | ||
+ | To quantify the effect of antifreeze proteins on ice formation, we created a specific assay called "I Said Freeze" (ISF). AFPs can be characterized in multiple ways, one of which is thermal hysteresis (TH). TH is the difference of the freezing and melting temperature of existing ice crystals in solution. Below the freezing point temperature, ice crystals grow, and above the melting point, ice crystals shrink. Between these two temperatures, ice crystals neither grow or shrink. | ||
<br> | <br> | ||
+ | <br> | ||
+ | To measure the TH, we need to measure the melting and freezing temperatures. We defined the melting temperature as the temperature at which there is only one ice crystal left and the freezing temperature as the moment at which the single ice crystal is growing rapidly and constantly. To see these ice crystals, we needed precise control of the temperature under a microscope. We therefore created Frozone (add link to wiki), a precise cooling device. | ||
<br> | <br> | ||
<br> | <br> | ||
+ | To have more precision, we used a microscope with a camera linked to a computer and our software to record ice crystal formation in our solution and follow the temperature at the same time. This allowed us to replay the experiment frame by frame to determine the precise temperatures. | ||
<br> | <br> | ||
− | < | + | <br> |
+ | We measured TH changes due to FfIBP using the ISF assay and found a TH of around 3K at a concentration of 200µM of FfIBP. The graph (Fig.3) shows how the TH is concentration dependent. | ||
+ | <br><br> | ||
+ | From these results, we can conclude that our antifreeze protein is functional, as we have successfully measured its TH value at two distinct concentrations. | ||
+ | <br><br><br><br> | ||
+ | </p> | ||
<h3>FDT Assay</h3> | <h3>FDT Assay</h3> | ||
+ | [[File:T--UNILausanne--FDT_GRAPH.png|400px|thumb|right|'''Figure 4:''' Our AFP solutions protect plants from frost damage. The graph shows mean +/- standard deviation. Percentage of Damage on Arabidopsis thaliana leaves measured after overnight incubation in a climatic chamber at -5ºC. Plants were immersed in phosphate-buffered saline (PBS) liquid solution supplemented with two different AFPs: FfIBP [200 µM] (n=13) and RiAFP [50 µM] (n=17) and a mix of them (RiAFP + FfIBP) (n=15) at a concentration of 25 µM and 100 µM, respectively. These solutions were compared with the control in which the plants were immersed in the PBS buffer without any AFP (buffer) (n=17). | ||
+ | The damage ratio is significantly different between the buffer and all AFPs. ANOVA, * significant at p<0.05.Damage ratio between the different AFPs are not significant.]] | ||
+ | <p style="margin-right:2.5em;" align="justify"> | ||
+ | To measure how FfIBP will affect damage on plants during frost conditions, we developed the Frost Damage Treatment (FDT) assay. We immersed Arabidopsis thaliana (Wild-type) in a buffer containing the antifreeze protein and then placed the plants in a thermal chamber at -5°C overnight. The following day we stained the plants with Trypan blue, which colors dead cells with a pierced membrane in blue. In our case, the stain highlighted the cells damaged by the ice crystals. | ||
+ | <br> | ||
+ | After the staining, we cut all the leaves of our plants and placed them on microscope slides to observe them under a microscope. We took pictures of all the leaves and analyzed them with our algorithm VISISION (add link to wiki) to obtain the percentage of damaged area on every leaf. Finally, we processed all the data. | ||
+ | <br> | ||
+ | <br> | ||
+ | We used the FDT assay to analyze if FfIBP can reduce frost damage on plants. We immersed Arabidopsis thaliana in different solutions: | ||
+ | <ul> | ||
+ | <li>A buffer solution without FfIBP, which served as a control to observe the damage on our plants without the protection of antifreeze proteins.</li> | ||
+ | <li>A buffer with FfIBP to observe how the protein reduces frost damage on our plants | ||
+ | After immersion of Arabidopsis thaliana in the various solutions, we placed the plants in a thermal chamber overnight, stained them the next day and analyzed them as previously described with our VISION algorithm.</li> | ||
+ | </ul> | ||
+ | <br><br> | ||
+ | Our results show that plants incubated in the buffer alone are significantly more damaged than those incubated in the AFP solutions, therefore confirming the protective effect of our AFPs. | ||
+ | </p> | ||
+ | <br><br><br><br><br><br><br><br><br><br><br> | ||
=Sequence and Features= | =Sequence and Features= | ||
<partinfo>BBa_K3782000 SequenceAndFeatures</partinfo> | <partinfo>BBa_K3782000 SequenceAndFeatures</partinfo> | ||
=References= | =References= | ||
{{reflist}} | {{reflist}} |
Latest revision as of 23:23, 21 October 2021
Flavobacterium frigoris strain PS1 ice-binding protein gene
FfIBP
FfIBP is a protein coding region that codes for an ice binding protein (IBP). IBPs, or more specifically antifreeze proteins (AFP), can bind to ice crystals and thereby prevent further ice growth. They are produced by organisms to survive in extremely cold environments. Activities of AFPs can be characterized by their thermal hysteresis (TH) or by their ice recrystallization inhibition (IRI). TH activity corresponds to the lowering of the freezing point without changing the melting point of a solution. IRI activity inhibits the growth of large ice crystals at the expense of smaller ones. The combination of these activities, which vary depending on the protein structure, prevents the freezing of body fluids and cell damage in organisms that live in environments with extremely cold temperatures.
Contents
Profile
Name | FfIBP |
Base pairs | 744 |
Number of amino acids | 247 |
Molecular weight | 25.35 kDa |
Origin | Flavobacterium frigoris strain PS1, synthetic |
Usage and Biology
The FfIBP protein coding region was used in the following composite parts (add links). It was expressed in E. coli strain BL21 (DE3) and purified. Various tests and assays were performed to characterize and verify the functionality of this ice-binding protein. FfIBP has moderate TH and IRI activity, and it can bind to ice crystals and inhibit their growth. The aim of our project was to use it on its own or in a mixture of other antifreeze proteins to develop a solution which could be applied on sensitive plant tissues and thereby protect crops from frost damage.
FfIBP is naturally produced by the Gram-negative Antarctic bacterium Flavobacterium frigoris PS1. Its TH activity is around 2.5 K at 50 µM[1].
Antifreeze proteins bind to ice crystals and inhibit their growth. Ice crystals don’t have the same molecular arrangement on each of its faces and certain AFPs tend to adsorb to the basal plane of the crystals whilst others tend to adsorb to the prism plane. Hyperactive AFPs can bind to every plane[2].
The mechanism by which AFPs suppress the freezing point of a solution still isn’t completely understood. Nevertheless, AFPs are believed to attach irreversibly to the plane of a growing ice crystal. As the protein adsorbs to the growing ice plane, growth at the site is suppressed, producing bulges in between the adsorbed proteins[3]. Due to the Gibbs-Thomson effect, the freezing point will be depressed[4].
Characterization
To reduce frost damage during late spring freeze, we wanted to develop a solution containing antifreeze proteins, which bind to ice crystals and inhibit their growth. FfIBP was therefore chosen as one of three AFPs, cloned and expressed in E. coli BL21 (DE3), and finally purified using a His-tag affinity column and gel filtration.
Two different vectors were used for cloning: pET-17b and pColdI, containing a T7 promoter and a cold-shock protein A (cspA) promoter respectively.
Cloning

FfIBP was cloned into the pET-17b plasmid using restriction and ligation via HindIII and XhoI and Gibson Assembly. The vector includes an ampicillin resistance gene and a lac operator, which allows for IPTG induced expression. The final construct was checked with a 1% Agarose gel (Fig.1) and via sequencing.
FfIBP was cloned into the pColdI plasmid using restriction and ligation via NdeI and XhoI and Gibson Assembly. The vector includes an ampicillin resistance gene and a lac operator, which allows for IPTG induced expression. The final construct was checked with a 1% Agarose gel (Fig.1) and via sequencing.
Expression and Purification
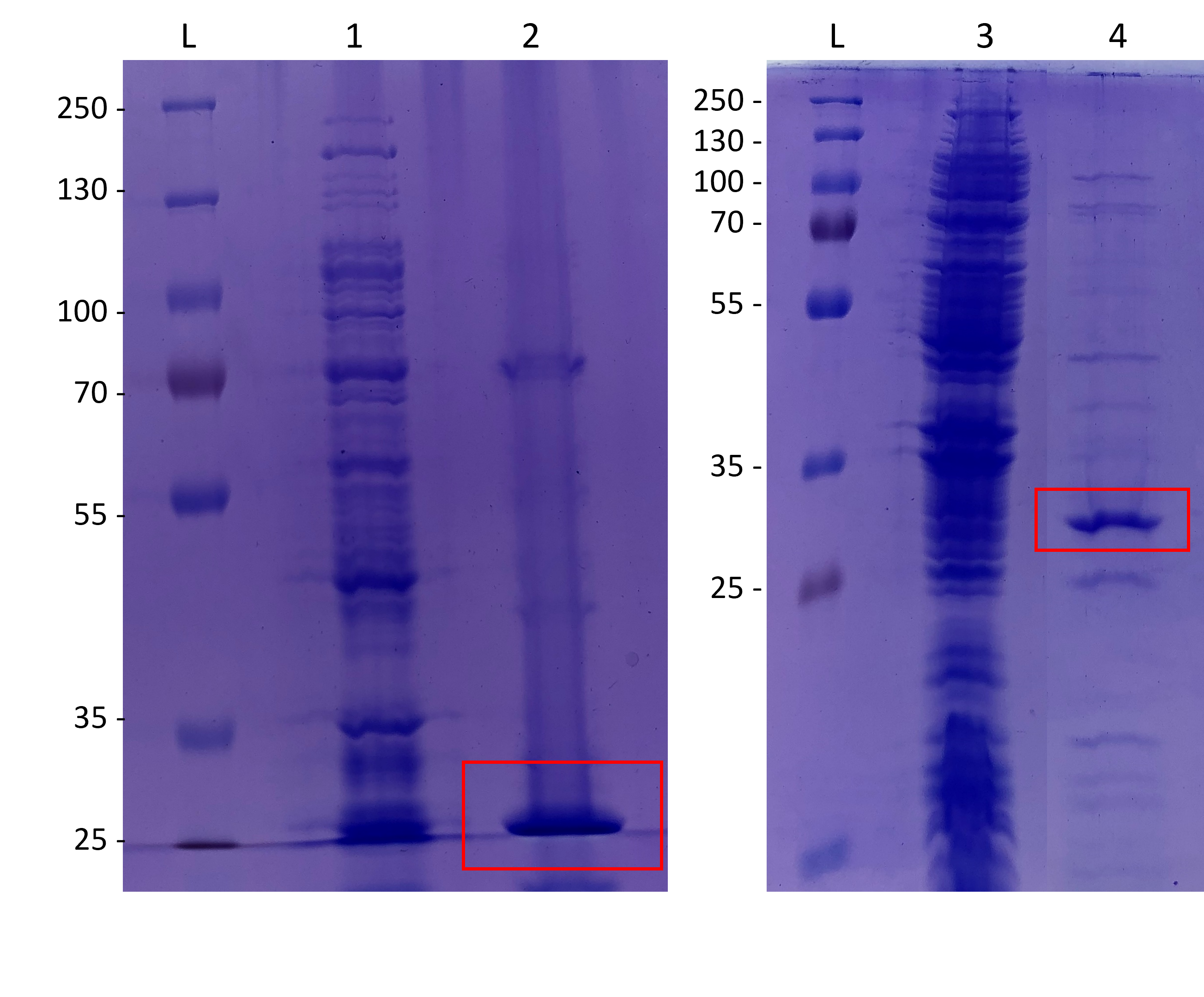
After we successfully transformed E. coli strain BL21 (DE3) with our pET-17b-FfIBP and pColdI-FfIBP constructs, we inoculated liquid cultures with LB and ampicillin. Before inducing expression in large volumes of liquid cultures, we first did small-scale test purifications to verify if the antifreeze proteins could be correctly purified. Next, we grew 1-liter liquid cultures at 37°C until an OD600 of 0.6 was reached. We then placed the culture in a cooling incubator at 15°C for 30 minutes. We added IPTG 1mM and induced expression at 15°C for 24 hours. Afterwards, we removed a small volume of culture to run a total cell SDS-PAGE (15%) of E. coli cultures stained with Coomassie blue and to verify that the antifreeze protein was correctly overexpressed. The remaining culture was centrifuged, and the cell pellet was resuspended in Cold Lysis Buffer. We sonicated the cells and centrifuged the cells once more to collect the supernatant, which we loaded into the His-tag affinity column. We eluted the antifreeze proteins which bound to the column and concentrated the solution to a smaller volume. We performed a gel filtration to further purify the protein and finally stored aliquots at -80°C.
We induced expression of FfIBP with IPTG and ran a total cell SDS-PAGE (15%) of the E. coli BL21 (DE3) cultures stained with Coomassie blue. We used two different vectors, pET-17b and pColdI, to compare how the promoters influenced expression efficiency. The overexpression was successful for FfIBP. We observed thicker bands that matched the weight of the protein. The pColdI vector worked better than the pET-17b vector, because the band appeared to be stronger for this first plasmid on the gel (Fig.2).
ISF Assay
To quantify the effect of antifreeze proteins on ice formation, we created a specific assay called "I Said Freeze" (ISF). AFPs can be characterized in multiple ways, one of which is thermal hysteresis (TH). TH is the difference of the freezing and melting temperature of existing ice crystals in solution. Below the freezing point temperature, ice crystals grow, and above the melting point, ice crystals shrink. Between these two temperatures, ice crystals neither grow or shrink.
To measure the TH, we need to measure the melting and freezing temperatures. We defined the melting temperature as the temperature at which there is only one ice crystal left and the freezing temperature as the moment at which the single ice crystal is growing rapidly and constantly. To see these ice crystals, we needed precise control of the temperature under a microscope. We therefore created Frozone (add link to wiki), a precise cooling device.
To have more precision, we used a microscope with a camera linked to a computer and our software to record ice crystal formation in our solution and follow the temperature at the same time. This allowed us to replay the experiment frame by frame to determine the precise temperatures.
We measured TH changes due to FfIBP using the ISF assay and found a TH of around 3K at a concentration of 200µM of FfIBP. The graph (Fig.3) shows how the TH is concentration dependent.
From these results, we can conclude that our antifreeze protein is functional, as we have successfully measured its TH value at two distinct concentrations.
FDT Assay

To measure how FfIBP will affect damage on plants during frost conditions, we developed the Frost Damage Treatment (FDT) assay. We immersed Arabidopsis thaliana (Wild-type) in a buffer containing the antifreeze protein and then placed the plants in a thermal chamber at -5°C overnight. The following day we stained the plants with Trypan blue, which colors dead cells with a pierced membrane in blue. In our case, the stain highlighted the cells damaged by the ice crystals.
After the staining, we cut all the leaves of our plants and placed them on microscope slides to observe them under a microscope. We took pictures of all the leaves and analyzed them with our algorithm VISISION (add link to wiki) to obtain the percentage of damaged area on every leaf. Finally, we processed all the data.
We used the FDT assay to analyze if FfIBP can reduce frost damage on plants. We immersed Arabidopsis thaliana in different solutions:
- A buffer solution without FfIBP, which served as a control to observe the damage on our plants without the protection of antifreeze proteins.
- A buffer with FfIBP to observe how the protein reduces frost damage on our plants After immersion of Arabidopsis thaliana in the various solutions, we placed the plants in a thermal chamber overnight, stained them the next day and analyzed them as previously described with our VISION algorithm.
Our results show that plants incubated in the buffer alone are significantly more damaged than those incubated in the AFP solutions, therefore confirming the protective effect of our AFPs.
Sequence and Features
- 10INCOMPATIBLE WITH RFC[10]Illegal PstI site found at 269
Illegal PstI site found at 350
Illegal PstI site found at 530 - 12INCOMPATIBLE WITH RFC[12]Illegal PstI site found at 269
Illegal PstI site found at 350
Illegal PstI site found at 530 - 21COMPATIBLE WITH RFC[21]
- 23INCOMPATIBLE WITH RFC[23]Illegal PstI site found at 269
Illegal PstI site found at 350
Illegal PstI site found at 530 - 25INCOMPATIBLE WITH RFC[25]Illegal PstI site found at 269
Illegal PstI site found at 350
Illegal PstI site found at 530 - 1000COMPATIBLE WITH RFC[1000]
References
Template:Reflist- ↑ Do H, Lee JH, Lee SG, Kim HJ. Crystallization and preliminary X-ray crystallographic analysis of an ice-binding protein (FfIBP) from Flavobacterium frigoris PS1. Acta Crystallogr Sect F Struct Biol Cryst Commun [Internet]. 2012 Jul [cited 2021 Jul 7];68(Pt 7):806. Available from: /pmc/articles/PMC3388927/
- ↑ Olijve LLC, Meister K, DeVries AL, Duman JG, Guo S, Bakker HJ, et al. Blocking rapid ice crystal growth through nonbasal plane adsorption of antifreeze proteins. Proc Natl Acad Sci [Internet]. 2016 Apr 5 [cited 2021 Oct 10];113(14):3740–5. Available from: https://www.pnas.org/content/113/14/3740
- ↑ LM S, AV T. Kinetic pinning and biological antifreezes. Phys Rev Lett [Internet]. 2004 Sep 17 [cited 2021 Oct 10];93(12). Available from: https://pubmed.ncbi.nlm.nih.gov/15447309/
- ↑ Pereyra RG, Szleifer I, Carignano MA. Temperature dependence of ice critical nucleus size. J Chem Phys [Internet]. 2011 Jul 21 [cited 2021 Oct 10];135(3):034508. Available from: https://aip.scitation.org/doi/abs/10.1063/1.3613672